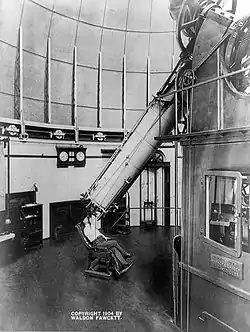
What is “the “old-fashioned” spirit of real-time visual astronomy”?[1] “I think everyone can conjure up a mental image of astronomers at every level and place in history, gazing through the eyepieces of their telescopes at sights far away - true visual astronomy.”[1]
Radiation
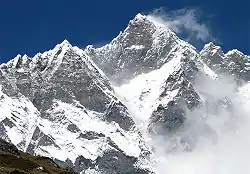
White is the color of fresh milk and snow.[2][3] It is the color the human eye sees when it looks at light which contains all the wavelengths of the visible spectrum, at full brightness and without absorption. It does not have any hue.[4]
"de la couleur de la neige, du lait. Lumiere resultant de la combinaison de toutes les couleurs du spectre solaire."[5] (of the color of snow, of milk. Light resulting from the combination of all the colors of the solar spectrum.)
Lights
Def. the natural medium emanating from the sun and other very hot sources (now recognised as electromagnetic radiation with a wavelength of 400-750 nm), within which vision is possible is called light.
Def. to shine light on something is called illuminate.
Fluorescences
Fluorescence is the emission of light by a substance that has absorbed light or other electromagnetic radiation. It is a form of luminescence. In most cases, the emitted light has a longer wavelength, and therefore lower energy, than the absorbed radiation. However, when the absorbed electromagnetic radiation is intense, it is possible for one electron to absorb two photons; this two-photon absorption can lead to emission of radiation having a shorter wavelength than the absorbed radiation. The emitted radiation may also be of the same wavelength as the absorbed radiation, termed "resonance fluorescence".[6]
The most striking examples of fluorescence occur when the absorbed radiation is in the ultraviolet region of the spectrum, and thus invisible to the human eye, and the emitted light is in the visible region.
The common fluorescent lamp relies on fluorescence. Inside the glass tube is a partial vacuum and a small amount of mercury. An electric discharge in the tube causes the mercury atoms to emit ultraviolet light. The tube is lined with a coating of a fluorescent material, called the phosphor, which absorbs the ultraviolet and re-emits visible light. Fluorescent lighting is more energy-efficient than incandescent lighting elements. However, the uneven spectrum of traditional fluorescent lamps may cause certain colors to appear different than when illuminated by incandescent light or daylight. The mercury vapor emission spectrum is dominated by a short-wave UV line at 254 nm (which provides most of the energy to the phosphors), accompanied by visible light emission at 436 nm (blue), 546 nm (green) and 579 nm (yellow-orange). These three lines can be observed superimposed on the white continuum using a hand spectroscope, for light emitted by the usual white fluorescent tubes. These same visible lines, accompanied by the emission lines of trivalent europium and trivalent terbium, and further accompanied by the emission continuum of divalent europium in the blue region, comprise the more discontinuous light emission of the modern trichromatic phosphor systems used in many compact fluorescent lamp and traditional lamps where better color rendition is a goal.[7]
Luminosities
Def. emitting light is called luminous.
Def. the measure of the power of light as perceived by the human eye, in proportion to the power at each wavelength is called the luminous flux.
Def. the measure of the total power of electromagnetic radiation is called radiant flux, or radiant power.
Limiting the electromagnetic radiation to the visible may be called the apparent radiant flux.
Def. a measure of the power emitted by a source of light; equal to the flux density per solid angle is called a luminous intensity.
Def. the radiant energy of the visible portion of a spectrum of light is called the luminous energy.
Def. a derived SI unit of luminous energy is called a lumen second, or a lumen-second.
Def. the total amount of visual, or visible light only electromagnetic energy emitted by a star or other astronomical object in SI units of joules per second, which are watts is called apparent luminosity.
Def. the ratio of luminous flux to radiant flux at the same wavelength is called luminosity.
Def. the rate at which a star radiates energy in all directions is called astronomical luminosity.
Skies
The overall theory of visual astronomy consists of three fundamental parts:
- the derivation of visual logical laws,
- the definitions of visual natural entities, and
- the definition of the visual sky (and associated realms).
Def. a visual or visible "expanse of space that seems to be [overhead] like a dome"[8] is called a visual sky, or visible sky.
Even in day light, the sky may seem absent of objects if a nearby source tends to overwhelm other luminous objects.
Naked eye astronomy
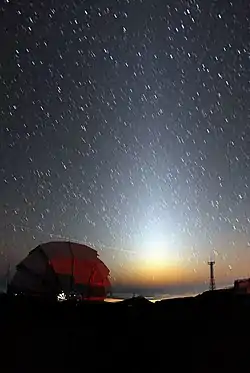
The visibility of astronomical objects is strongly affected by light pollution. Even a few hundred kilometers away from a metropolitan area where the sky can appear to be very dark, it is still the residual light pollution that sets the limit on the visibility of faint objects. For most people, this is likely to be the best observing conditions within their reach. Under such "typical" dark sky conditions, the naked eye can see stars with an apparent magnitude up to +6m. Under perfect dark sky conditions where all light pollution is absent, stars as faint as +8m might be visible.[9] The angular resolution of the naked eye is about 1′; however, some people have sharper vision than that. There is anecdotal evidence that people had seen the Galilean moons of Jupiter before telescopes were invented.[10] Of similar magnitude, Uranus and Vesta had most probably been seen but could not be recognized as planets because they appear so faint even at maximum brightness that their motion could not be detected.
"According to Gruson and Brugsch the Egyptians were acquainted with, and even worshipped, the zodiacal light from the very earliest times, as a phenomenon visible throughout the East before sunrise and after sunset. It was described as a glowing sheaf or luminous pyramid perpendicular to the horizon in summer, and inclined more or less during the winter. Indeed the Egyptians represented the zodiacal light under the form of a triangle which sometimes stood upright and at other times was inclined."[11]
Whites
.JPG.webp)
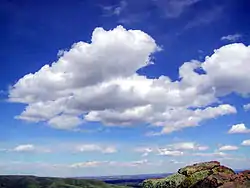
The white cliffs shown in the image at right are made of chalk, or calcium carbonate.
White light reflected off objects can be seen when no part of the light spectrum is reflected significantly more than any other and the reflecting material has a degree of diffusion. People see this when transparent fibers, particles, or droplets are in a transparent matrix of a substantially different refractive index. Examples include classic "white" substances such as sugar, foam, pure sand or snow, cotton, clouds, and milk. Crystal boundaries and imperfections can also make otherwise transparent materials white, as in the milky quartz or the microcrystalline structure of a seashell.
Cumulus clouds look white because the water droplets reflect and scatter the sunlight without absorbing other colors.
White light is generated by the sun, by stars, or by earthbound sources such as incandescent lamps, fluorescent lamps and white LEDs.
Blacks
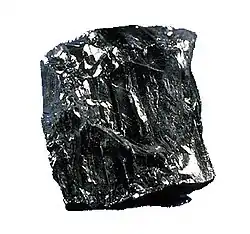
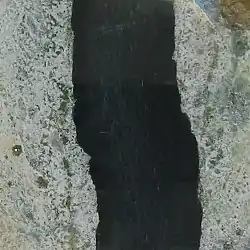
Black is the color of coal, ebony, and of outer space. It is the darkest color, the result of the absence of or complete absorption of light. It is the opposite of white and often represents darkness in contrast with light.[12][13][14]
"Opposite to white: colourless from the absence or complete absorption of light. Also, so near this as to have no distinguishable colour, very dark."[12]
Black is "[t]he darkest color".[13]
"Se dit de la couleur la plus foncée, due à l'absence ou à l'absorption totale des rayons lumineux."[14]("said of the very darkest color, due to the absence or complete absorption of all rays of light.")
Grays

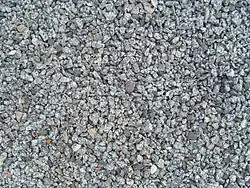

Grey or gray is an intermediate color between black and white, a neutral or achromatic color, meaning literally a color "without color." [15] It is the color of a cloud-covered sky, of ash and of lead.[16]
The first image at right shows some red pebbles among gray pebbles, which are all the same rock type.
The first image at left shows the various shades of grey.
The second image at left of gray clay shows the cracking from water loss.
Albedos
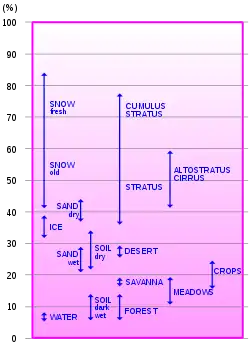
Def. the fraction of incident light or radiation reflected by a surface or body, commonly expressed as percentage is called albedo.
Albedo, or reflection coefficient, derived from Latin albedo "whiteness" (or reflected sunlight), in turn from albus "white", is the diffuse reflectivity or reflecting power of a surface. It is defined as the ratio of reflected radiation from the surface to incident radiation upon it. Being a dimensionless fraction, it may also be expressed as a percentage, and is measured on a scale from zero for no reflecting power of a perfectly black surface, to 1 for perfect reflection of a white surface.
Albedo depends on the frequency of the radiation. When quoted unqualified, it usually refers to some appropriate average across the spectrum of visible light. In general, the albedo depends on the directional distribution of incoming radiation. Exceptions are Lambertian surfaces, which scatter radiation in all directions according to a cosine function, so their albedo does not depend on the incident distribution. In practice, a bidirectional reflectance distribution function (BRDF) may be required to characterize the scattering properties of a surface accurately, although the albedo is a very useful first approximation.
Albedos of typical materials in visible light range from up to 0.9 for fresh snow, to about 0.04 for charcoal, one of the darkest substances. Deeply shadowed cavities can achieve an effective albedo approaching the zero of a black body. When seen from a distance, the ocean surface has a low albedo, as do most forests, whereas desert areas have some of the highest albedos among landforms. Most land areas are in an albedo range of 0.1 to 0.4.[17] The average albedo of the Earth is about 0.3.[18] This is far higher than for the ocean primarily because of the contribution of clouds.
Two common albedos that are used in astronomy are the (V-band) geometric albedo (measuring brightness when illumination comes from directly behind the observer) and the Bond albedo (measuring total proportion of electromagnetic energy reflected). Their values can differ significantly.
Minerals
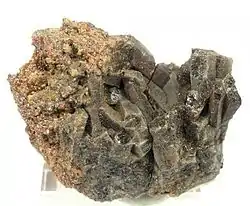
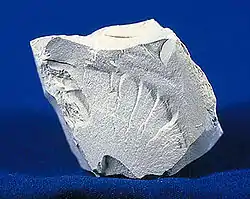
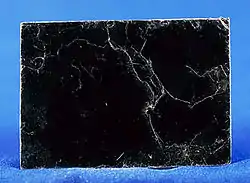
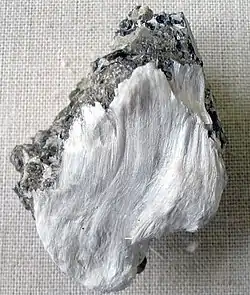
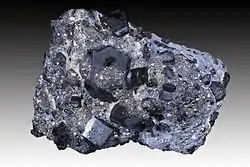
.JPG.webp)
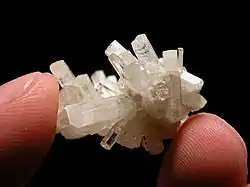
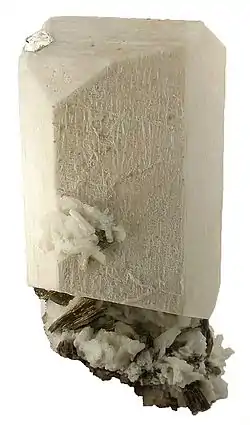
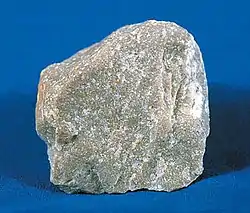
Beryl of various colors is found most commonly in granitic pegmatites, but also occurs in mica schists. Goshenite [a beryl clear to white cyclosilicate] is found to some extent in almost all beryl localities.
Quartzite (from German Quarzit[19]) is a hard, non-foliated metamorphic rock which was originally pure quartz sandstone.[20][21] Sandstone is converted into quartzite through heating and pressure usually related to tectonic compression within orogenic belts. Pure quartzite is usually white to gray, though quartzites often occur in various shades of pink and red due to varying amounts of iron oxide (Fe2O3). Other colors, such as yellow and orange, are due to other mineral impurities.
The great majority of silicates are oxides which comprise the majority of the earth's crust, as well as the other terrestrial planets, rocky moons, and asteroids. Sand, Portland cement, and thousands of minerals are examples of silicates.
Mineralogically, silicate minerals are divided according to structure of their silicate anion into the following groups:[22][23]
- Nesosilicates (lone tetrahedron) - [SiO4]4−, e.g. olivine, tephroite.
- Sorosilicates (double tetrahedra) - [Si2O7]6−, e.g. epidote, melilite group, leucophanite.
- Cyclosilicates (rings) - [SinO3n]2n−, e.g. tourmaline group.
- Inosilicates (single chain) - [SinO3n]2n−, e.g. pyroxene group.
- Inosilicates(double chain) - [Si4nO11n]6n−, e.g. amphibole group.
- Phyllosilicates (sheets) - [Si2nO5n]2n−, e.g. micas and clays like Kaolin.
- Tectosilicates (3D framework) - [AlxSiyO2(x+y)]x−, e.g. quartz, feldspars, zeolites.
Visions
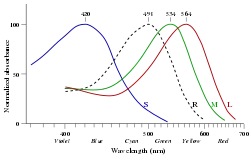
At right are normalized absorption spectra of the three human photopsins and of human rhodopsin.
Iodopsins ... are the photoreceptor proteins found in the cone cells of the [human] retina that are the basis of color vision. Iodopsins are very close analogs of the visual purple rhodopsin [R] that is used in night vision. Iodopsins consist of a protein called photopsin and a bound chromophore, retinal.
In humans there are three different iodopsins (rhodopsin analogs) that form the protein-pigment complexes photopsin I, II, and III. They are called erythrolabe, chlorolabe, and cyanolabe, respectively.[24] These photopsins have absorption maxima for yellowish-green (photopsin I), green (photopsin II), and bluish-violet light (photopsin III).
Color indexes
Class | B–V | V–R | Teff (K) |
---|---|---|---|
O5V | –0.33 | –0.15 | 42,000 |
B0V | –0.30 | –0.13 | 30,000 |
A0V | –0.02 | 0.02 | 9,790 |
F0V | 0.30 | 0.30 | 7,300 |
G0V | 0.58 | 0.50 | 5,940 |
K0V | 0.81 | 0.64 | 5,150 |
M0V | 1.40 | 1.28 | 3,840 |
"[T]he [visual] color index is a simple numerical expression that determines the color of an object, which in the case of a star gives its temperature. To measure the [visual] index, one observes the magnitude of an object successively through two different filters, such as ... B and V, where ... B is sensitive to blue light, and V is sensitive to visible (green-yellow) light ... The difference in magnitudes found with these filters is called the ... B–V color index ... The smaller the color index, the more blue (or hotter) the object is. Conversely, the larger the color index, the more red (or cooler) the object is. ... For comparison, the yellowish Sun has a B–V index of 0.656 ± 0.005,[26] while the bluish Rigel has a B–V of –0.03 (its B magnitude is 0.09 and its V magnitude is 0.12, B–V = –0.03).[27]
Color indices of distant objects are usually affected by interstellar extinction —i.e. they are redder than those of closer stars. The amount of reddening is characterized by color excess, defined as the difference between the Observed color index and the Normal color index (or Intrinsic color index), the hypothetical true color index of the star, unaffected by extinction. For example, we can write it for the B-V color:
Visible bands
Molecules of "[l]arge polycyclic aromatic hydrocarbons (PAH) ... or their ions are also attractive candidates for the carriers of the diffuse interstellar bands in the visible (DIBs) [because]
- they have optically active transitions in the visible;
- they can survive the UV photons in the diffuse interstellar medium; [and]
- they are the most abundant among the detected molecular species after H2 and CO."[28]
Visible spectrum
![]() sRGB rendering of the spectrum of visible light | ||
---|---|---|
Color | Frequency | Wavelength |
violet | 668–789 THz | 380–450 nm |
blue | 631–668 THz | 450–475 nm |
cyan | 606–630 THz | 476–495 nm |
green | 526–606 THz | 495–570 nm |
yellow | 508–526 THz | 570–590 nm |
orange | 484–508 THz | 590–620 nm |
red | 400–484 THz | 620–750 nm |
The visible spectrum is the portion of the electromagnetic spectrum that is visible to (can be detected by) the human eye. Electromagnetic radiation in this range of wavelengths is called visible light or simply light. A typical human eye will respond to wavelengths from about 390 to 750 nm.[29] In terms of frequency, this corresponds to a band in the vicinity of 400–790 THz. A light-adapted eye generally has its maximum sensitivity at around 555 nm (540 THz), in the green region of the optical spectrum (see: luminosity function).
Below are the visible emission spectra from the first eight elements of the periodic table.
Theoretical visual astronomy
Def. the ratio of the flux reflected to that incident on a surface is called a reflectance.
According to the CIE (the International Commission on Illumination),[30] reflectivity is distinguished from reflectance by the fact that reflectivity is a value that applies to thick reflecting objects.[31] When reflection occurs from thin layers of material, internal reflection effects can cause the reflectance to vary with surface thickness. Reflectivity is the limit value of reflectance as the sample becomes thick; it is the intrinsic reflectance of the surface, hence irrespective of other parameters such as the reflectance of the rear surface. Another way to interpret this is that the reflectance is the fraction of electromagnetic power reflected from a specific sample, while reflectivity is a property of the material itself, which would be measured on a perfect machine if the material filled half of all space.[32]
Specular reflection is the mirror-like reflection of light (or of other kinds of wave) from a surface, in which light from a single incoming direction (a ray) is reflected into a single outgoing direction.
Specular reflection is distinct from diffuse reflection, where incoming light is reflected in a broad range of directions.
Def. the condition of being specular, or the degree to which something is specular is called specularity.
Specularity refers the smoothness of a surface. A highly specular surface is very smooth.
It has been shown that for many applications involving terrestrial albedo, the albedo at a particular solar zenith angle can reasonably be approximated by the proportionate sum of two terms: the directional-hemispherical reflectance at that solar zenith angle, , and the bi-hemispherical reflectance, the proportion concerned being defined as the proportion of diffuse illumination . Bold added.
Albedo may be given by:
Directional-hemispherical reflectance is sometimes referred to as black-sky albedo and bi-hemispherical reflectance as white-sky albedo. These terms are important because they allow the albedo to be calculated for any given illumination conditions from a knowledge of the intrinsic properties of the surface.[33]
In detailed studies, the directional reflectance properties of astronomical bodies are often expressed in terms of the five Hapke parameters which semi-empirically describe the variation of albedo with phase angle, including a characterization of the opposition effect of regolith surfaces.
The correlation between astronomical (geometric) albedo, absolute magnitude and diameter is:[34]
where is the astronomical albedo, is the diameter in kilometers, and is the absolute magnitude.
Sources
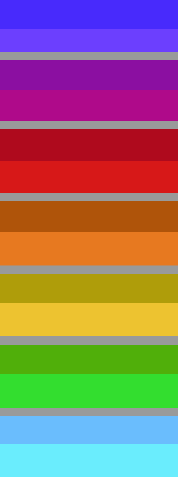
The Abney effect describes the perceived hue shift that occurs when white light is added to a monochromatic light source.[35]
The addition of white light will cause a desaturation of the monochromatic source, as perceived by the human eye. However, a less intuitive effect of the white light addition that is perceived by the human eye is the change in the apparent hue. This hue shift is physiological rather than physical in nature.
A white light source is created by the combination of red light, blue light, and green light.
The term hue discrimination is used to describe the change in wavelength that must be obtained in order for the eye to detect a shift in hue. An expression λ + Δλ defines the required wavelength adjustment that must take place.[36] A less than two nanometer change in wavelength causes most spectral colors to appear to take on a different hue. However, for blue light and red light, a much larger wavelength shift must occur in order for a person to be able to identify a difference in hue.
A white point (often referred to as reference white or target white in technical documents) is a set of tristimulus values or chromaticity coordinates that serve to define the color "white" in image capture, encoding, or reproduction.[37] Depending on the application, different definitions of white are needed to give acceptable results. For example, photographs taken indoors may be lit by incandescent lights, which are relatively orange compared to daylight. Defining "white" as daylight will give unacceptable results when attempting to color-correct a photograph taken with incandescent lighting.
Continua
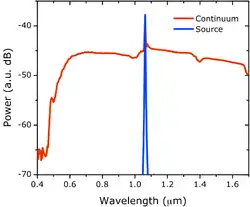
Black and white images are not ordinarily starkly contrasted black and white but combine black and white in a continuum producing a range of shades of gray.
In physics, a continuous spectrum usually means a set of values for some physical quantity (such as energy or wavelength) that is best described as an interval of real numbers. It is opposed to discrete spectrum, a set of values that is discrete in the mathematical sense, where there is a positive gap between each value and the next one.
At left is a continuous spectrum from a deuterium lamp. The tall sharp peaks are discrete emissions and the continuous part is the smoothly varying part between the peaks. The smaller peaks and valleys may be due to measurement errors rather than discrete spectral lines.
"[W]ith Scorpius X-1 ... the visible continuum is roughly what would be expected from a hot plasma fitting the observed X-ray flux.[38] The plasma could be a coronal cloud of a central object or a transient plasma, where the energy source is unknown, but could be related to the idea of a close binary.[38]
A spectrum (plural spectra or spectrums[39]) is a condition that is not limited to a specific set of values but can vary infinitely within a continuum.
In the continuum of colors of visible light [green] is located between yellow and blue.
Continuum light is linearly polarized at different locations across the face of the Sun (limb polarization) though taken as a whole, this polarization cancels.
In optics, a supercontinuum is formed when a collection of nonlinear processes act together upon a pump beam in order to cause severe spectral broadening of the original pump beam. The result is a smooth spectral continuum.
The figure at right shows a typical supercontinuum spectrum about an emission-line source. The blue line shows the spectrum of the source launched into a photonic crystal fiber while the red line shows the resulting supercontinuum spectrum generated after propagating through the fiber.
The inverse Raman effect[40] in optics ... which deals with the properties and behavior of light) is a form of Raman scattering.
If a material is simultaneously irradiated by intense monochromatic light of frequency νL (typically a laser beam) and light of a continuum of higher frequencies, among the possibilities for light scattering are scattering:
- from the monochromatic beam at νL to the continuum at νL+νM (anti-Stokes Raman scattering)
- from the continuum at νL+νM to the monochromatic beam at νL (Stokes Raman scattering)
where νM is a Raman frequency of the material.
The strength of these two scatterings depends (among other things) on the energy levels of the material, their occupancy, and the intensity of the continuum. In some circumstances Stokes scattering can exceed anti-Stokes scattering; in these cases the continuum (on leaving the material) is observed to have an absorption line (a dip in intensity) at νL+νM. This phenomenon is referred to as the inverse Raman effect; the application of the phenomenon is referred to as inverse Raman spectroscopy, and a record of the continuum is referred to as an inverse Raman spectrum.
Both absorption from a continuum of higher frequencies and absorption from a continuum of lower frequencies [can occur]. Absorption from a continuum of lower frequencies will not be observed if the Raman frequency of the material is vibrational in origin and if the material is in thermal equilibrium.
Reverberation mapping is an astrophysical technique for measuring the structure of the broad emission-line region (BLR) around a supermassive black hole at the center of an active galaxy and estimating the hole's mass. It is considered a "primary" mass estimation technique, i.e., the mass is measured directly from the motion that its gravitational force induces in the nearby gas.[41]
The black hole mass is measured from the formula
In this formula, ΔV is the rms velocity of gas moving near the black hole in the broad emission-line region, measured from the Doppler broadening of the gaseous emission lines; RBLR is the radius of the broad-line region; G is the constant of gravitation; and f is a poorly-known "form factor" that depends on the shape of the BLR.
The biggest difficulty with applying this formula is the measurement of RBLR. One standard technique[42] is based on the fact that the emission-line fluxes vary strongly in response to changes in the continuum, i.e., the light from the accretion disk near the black hole ("reverberation"). Furthermore, the emission-line response is found to be delayed with respect to changes in the continuum. Assuming that the delay is due to light travel times, the size of the broad emission-line region can be measured.
Only a small handful of AGN (less than 40) have been accurately "mapped" in this way. An alternative approach is to use an empirical correlation between RBLR and he continuum luminosity.[41]
Another uncertainty is the value of f. In principle, the response of the BLR to variations in the continuum could be used to map out the three-dimensional structure of the BLR. In practice, the amount and quality of data required to carry out such a deconvolution is prohibitive. Until about 2004, f was estimated ab initio based on simple models for the structure of the BLR. More recently, the value of f has been determined so as to bring the M-sigma relation for active galaxies into the best possible agreement with the M-sigma relation for quiescent galaxies.[41] When f is determined in this way, reverberation mapping becomes a "secondary", rather than "primary," mass estimation technique.
Backgrounds
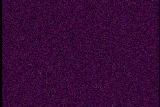
The diffuse extragalactic background light (EBL) is all the accumulated radiation in the Universe due to star formation processes, plus a contribution from active galactic nuclei (AGNs). This radiation covers the wavelength range between ~ 0.1-1000 microns (these are the ultraviolet, optical, and infrared regions of the electromagnetic spectrum). The EBL is part of the diffuse extragalactic background radiation (DEBRA), which by definition covers the overall electromagnetic spectrum. After the cosmic microwave background, the EBL produces the second-most energetic diffuse background, thus being essential for understanding the full energy balance of the universe.
The observed radiation density (the sky brightness of extragalactic background light) can be independent of finiteness of the Universe.
In astronomy, background commonly refers to the incoming light from an apparently empty part of the night sky.
In astronomy, background noise is electromagnetic radiation from the sky with no discernible source.
Even if no visible astronomical objects are present in given part of the sky, there always is some low luminosity present, due mostly to light diffusion from the atmosphere (diffusion of both incoming light from nearby sources, and of man-made Earth sources like cities). In the visible band, luminosity level is around the 22nd magnitude per square-arcsecond: a very low level, but anyway well within the limits of the current generation of telescopes.
There are several sources which contribute to the brightness of the (night) sky. Some of these are instrumental, or due to the presence of the atmosphere (like the airglow), in the case of ground based instruments. Even if we able to minimize the effect of instrumental and atmospherical components (e.g. using a spacecraft), there are still several astrophysical components contributing to the sky background: these could be sets of point sources like faint asteroids, Galactic stars and far away galaxies, as well as diffuse sources like dust in the Solar System, in the Milky Way, and in the intergalactic space. The actual importance of a specific component depends mostly of the wavelength of the measurement. The uncertainty (or noise) of the measurements caused by the astrophysical components of the sky background is called confusion noise.
Visual snow is a transitory or persisting snow or television-like static in parts or the whole of [the] visual fields, especially against dark backgrounds. It is much like camera noise in low light conditions. The visual frame at right shows an example of visual snow.
In astronomical CCD technology, background is usually referred to the overall optical "noise" of the system, that is, the incoming light on the CCD sensor in absence of light sources. This background can originate from electronic noise in the CCD, from not-well-masked lights nearby the telescope, and so on. An exposure on an empty patch of the sky is also called a background, and is the sum of the system background level plus the sky's one.
A background frame is often the first exposure in an astronomical observation with a CCD: the frame will then be subtracted from the actual observation result, leaving in theory only the incoming light from the astronomical object being observed.
Hydrogens

The Balmer series of emission lines from hydrogen occur in the visible spectrum of the Sun at: 397, 410, 434, 486, and 656 nm. Hα is the red line at the far right.
Helium

Helium was first detected as an unknown yellow spectral line signature in sunlight during a solar eclipse in 1868 by French astronomer Jules Janssen. Janssen is jointly credited with detecting the element along with Norman Lockyer during the solar eclipse of 1868, and Lockyer was the first to propose that the line was due to a new element, which he named.
Lithiums

"Violet satellite bands are caused by those lithium atoms which undergo an optical transition while a helium atom is nearby."[43]
Lithium I has an orange line at 610.3 nm. In some 824 red giant stars, the Li I 670.78 nm line was detected in several stars, "but only the five objects ... presented a strong line. Indeed, the Li subordinate line at 6103.6 Å was detected in these stars only."[44]
Berylliums

The visual spectrum for beryllium is missing characteristic lines in the yellow and orange.
Borons

Similar to beryllium, the visual spectrum of boron is missing lines in the yellow, but unlike it is also missing lines in the green and has a line in the orange.
Carbons

There is a C2 band at 619.1 nm.[45] Sometimes there is a hint of 13C12C at 618.8 nm.[45]
Carbon has a representative line in each of the major visual colors.
Nitrogens

"[T]he 5198-, 5201-A lines of nitrogen [occur] in the [Earth's] nightglow."[46]
Oxygens

"[A]irglow emissions [have been] measured by using vertical-viewing photometers [for the] O(1S) green line at 557.7 nm [with a] background at 566 nm"[47].
Fluorine
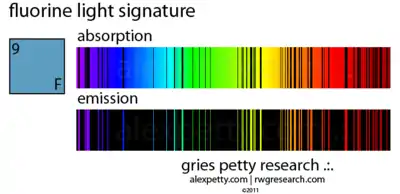
Fluorine has green emission lines that occur in plasmas at 526.83, 528.56, 529.76 and 530.27 nm from F VI.[48]
Fluorine has two emission lines that occur in plasmas at 479.132 and 479.45 nm from F IX.[48]
Fluorine has an emission line that occurs in plasmas at 429.92 nm from F II.[48]
Neon

There is a "bright yellow line of neon X [at] 5852.488 [Å, 585.2488 nm.]"[49]
Neon has two emission lines at 614.3 and 616.3 nm from Ne I.[50]
Absorption lines
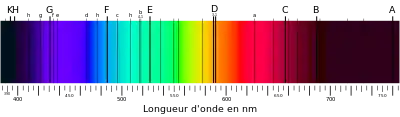
Fraunhofer's original (1817) designations of absorption lines in the solar spectrum
Letter | Wavelength (nm) | Chemical origin | Colour range |
---|---|---|---|
A |
759.37 |
atmospheric O2 |
dark red |
B |
686.72 |
atmospheric O2 |
red |
C |
656.28 |
hydrogen alpha |
red |
D1 |
589.59 |
neutral sodium |
red orange |
D2 |
589.00 |
neutral sodium |
yellow |
E |
526.96 |
neutral iron |
green |
F |
486.13 |
hydrogen beta |
cyan |
G |
431.42 |
CH molecule |
blue |
H |
396.85 |
ionised calcium |
dark violet |
K |
393.37 |
ionised calcium |
dark violet" |
Fraunhofer line 'A' is an optical astronomy absorption line, not a visual astronomy absorption line.
Planetary sciences
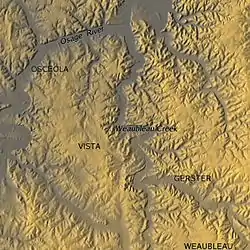
"The Weaubleau structure is a probable meteorite impact site in western Missouri near the towns of Gerster, Iconium, Osceola, and Vista. It is believed to have been caused by a 1200-ft (366 m) meteoroid between 330 and 335 million years ago[51] during the late Mississippian Period (Latest Osagean to Earliest Meramecian). It is listed by the Impact Field Studies Group as a "probable" impact structure.[52]
The structure consists of an area of severe structural deformity and extensive brecciation that was poorly understood and had been thought to be the result of either thrusting over a dome[53] or a cryptoexplosive event.[54] A 19-km-diameter (12 mi) circular structure was discovered ... through examination of digital elevation data.[55]
Because the site was covered by later Pennsylvanian Period sediments, and only partially exposed to erosion relatively recently, its structure is well preserved, and its age can be determined with fair accuracy. It is one of a series of known or suspected impact sites along the 38th parallel in the states of Illinois, Missouri, and Kansas.
The Weaubleau structure is one of the fifty largest known impact craters on earth and the fourth largest in the United States. The three larger ones in the US either have been glaciated and buried (Manson crater), are under water (Chesapeake Bay crater), or have been subjected to orogeny (Beaverhead crater). Therefore the Weaubleau structure is the largest exposed untectonized impact crater in the US.[55]
Meteors
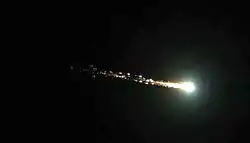
Meteors become visible between about 75 to 120 kilometers (34 - 70 miles) above the Earth. They disintegrate at altitudes of 50 to 95 kilometers (31-51 miles). Most meteors are observed at night, when darkness allows fainter objects to be recognized. Most meteors glow for about a second.
A fireball is a brighter-than-usual meteor. The International Astronomical Union defines a fireball as "a meteor brighter than any of the planets" (magnitude −4 or greater).[56] The International Meteor Organization (an amateur organization that studies meteors) has a more rigid definition. It defines a fireball as a meteor that would have a magnitude of −3 or brighter if seen at zenith. This definition corrects for the greater distance between an observer and a meteor near the horizon. For example, a meteor of magnitude −1 at 5 degrees above the horizon would be classified as a fireball because if the observer had been directly below the meteor it would have appeared as magnitude −6.[57] For 2011 there are 4589 fireballs records at the American Meteor Society.[58]
Def. a fireball reaching magnitude −14 or brighter.[59] is called a bolide.
Def. a fireball reaching an magnitude −17 or brighter is called a superbolide.
At right is a cell phone camera image of the green fireball over San Mateo, California, that left meteorite fragments. "The asteroid entered at a speed of 14 km/s, typical but on the slow side of other meteorite falls for which orbits were determined. ... The orbit in space is also rather typical: perihelion distance close to Earth's orbit (q = 0.987 AU) and a low-inclination orbit (about 5 degrees). ... 2012, October 17 - At 7:44:29 pm PDT this evening, a bright fireball was seen in the San Francisco Bay Area."[60]
Venus
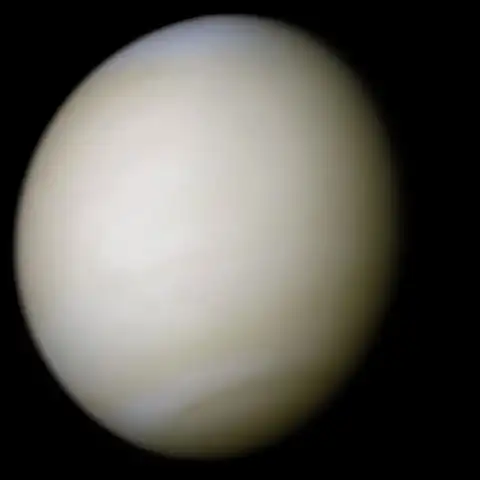
In visual astronomy almost no variation or detail can be seen in the clouds. The surface is obscured by a thick blanket of clouds. Venus is shrouded by an opaque layer of highly reflective clouds of sulfuric acid, preventing its surface from being seen from space in visible light. It has thick clouds of sulfur dioxide. There are lower and middle cloud layers. The thick clouds consisting mainly of sulfur dioxide and sulfuric acid droplets.[61][62] These clouds reflect and scatter about 90% of the sunlight that falls on them back into space, and prevent visual observation of the Venusian surface. The permanent cloud cover means that although Venus is closer than Earth to the Sun, the Venusian surface is not as well lit.
Earth
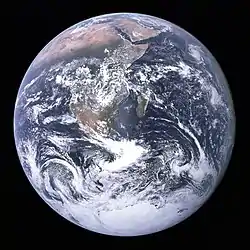
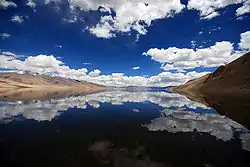
The average overall albedo of Earth, its planetary albedo, is 30 to 35%, because of the covering by clouds, but varies widely locally across the surface, depending on the geological and environmental features.[63]
Although the albedo–temperature effect is best known in colder, whiter regions on Earth, the maximum albedo is actually found in the tropics where year-round illumination is greater. The maximum is additionally in the northern hemisphere, varying between 3 and 12 degrees north.[64] The minima are found in the subtropical regions of the northern and southern hemispheres, beyond which albedo increases without respect to illumination.[64]
Deciduous trees have an albedo value of about 0.15 to 0.18 whereas coniferous trees have a value of about 0.09 to 0.15.[65]
The relative (generally warming) effect of albedo change and (cooling) effect of carbon sequestration on planting forests indicates that new forests in tropical and midlatitude areas tended to cool; new forests in high latitudes (e.g. Siberia) were neutral or perhaps warming.[66]
Cryoconite, powdery windblown dust containing soot, sometimes reduces albedo on glaciers and ice sheets.[67]
At small angles of incident light, waviness [of water] results in reduced reflectivity because of the steepness of the reflectivity-vs.-incident-angle curve and a locally increased average incident angle.[68]
"On any given day, about half of Earth is covered by clouds, which reflect more sunlight than land and water. Clouds keep Earth cool by reflecting sunlight, but they can also serve as blankets to trap warmth."[69]
As the second image on the right shows, specular reflection, or reflectance, occurs on Earth.
Moon
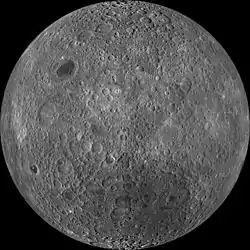
The overall albedo of the Moon is around 0.12, but it is strongly directional and non-Lambertian, displaying also a strong opposition effect.[70]
Mars

"This is the south polar cap of Mars as it appeared to the Mars Global Surveyor (MGS) Mars Orbiter Camera (MOC) on April 17, 2000. In winter and early spring, this entire scene would be covered by frost. In summer, the cap shrinks to its minimum size, as shown here. Even though it is summer, observations made by the Viking orbiters in the 1970s showed that the south polar cap remains cold enough that the polar frost (seen here as white) consists of carbon dioxide. Carbon dioxide freezes at temperatures around -125° C (-193° F). Mid-summer afternoon sunlight illuminates this scene from the upper left from about 11.2° above the horizon. Soon the cap will experience sunsets; by June 2000, this pole will be in autumn, and the area covered by frost will begin to grow. Winter will return to the south polar region in December 2000. The polar cap from left to right is about 420 km (260 mi) across."[71]
Enceladus
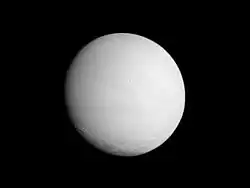
At right is Saturn's natural satellite, the rocky object, Enceladus.
Enceladus, a moon of Saturn, has one of the highest known albedos of any body in the Solar system, with 99% of EM radiation reflected.
"The Cassini spacecraft looks at a brightly illuminated Enceladus and examines the surface of the leading hemisphere of this Saturnian moon."[72]
"North on Enceladus (313 miles across, or 504 kilometers) is up and rotated 21 degrees to the right."[72]
"The image was taken in visible light with the Cassini spacecraft narrow-angle camera on Nov. 6, 2011. The view was obtained at a distance of approximately 67,700 miles (109,000 kilometers) from Enceladus and at a Sun-Enceladus-spacecraft, or phase, angle of 21 degrees. Image scale is 2,130 feet (649 meters) per pixel."[72]
Comets
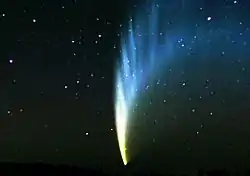
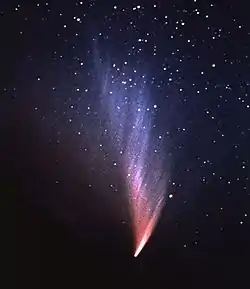
A typical comet nucleus has an albedo of 0.04.[73]
At left is an image of Comet West. "Comet West was a stunning sight in the predawn sky of March, 1976, bright with a tall and broad dust tail. ... [T]he comet [was] discovered on photographs taken in August 1975 by Richard West of the European Southern Observatory ... Comet West passed perihelion on February 25, 1976, at a distance of 0.20 a.u. [and] had reached about magnitude -3 at perihelion. Several observers saw it telescopically in daylight, and John Bortle observed it with the naked eye shortly before sunset. ... The following morning, March 7, ... It was brilliant, with a head as bright as Vega (which was nearly overhead) and a huge tail, about 20 degrees tall, straight near the bottom and bending to the left in its upper reaches. The comet quickly faded during March".[74]
Although many comets are photographed in black and white, not that many are actually only white but have colors. The image at right of McNaught Comet shows white and other colors, as does Comet West at left.
Eris
A notable high-albedo body is Eris, with an albedo of 0.96.[75]
Opticals

In popular culture optical astronomy encompasses a wide variety of observations via telescopes that are sensitive in the range of visible light. Scientists would call this visible-light astronomy. It includes imaging, where a picture of some sort is made of the object; photometry, where the amount of light coming from an object is measured, spectroscopy, where the distribution of that light with respect to its wavelength is measured, and polarimetry where the polarisation state of that light is measured.
Violets
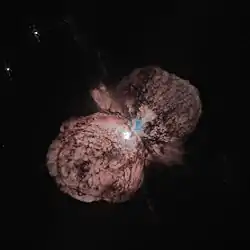
Violet astronomy is conducted over wavelengths between 380 and 450 nm.
Violet photographs of the planet Venus taken in 1927 “recorded two nebulous bright streaks, or bands, running ... approximately at right angles to the terminator” that may be from the upper atmosphere.[76]
"A definite color gradient is observed [in the small inner satellites of Jupiter], with the satellites closer to Jupiter being redder: the mean violet/green ratio (0.42/0.56 μm) decreases from Thebe to Metis. This ratio also is lower for the trailing sides of Thebe and Amalthea than for their leading sides."[77]
"The “Purple Haze” is a diffuse blueish/purple glow within a few arcseconds of the central star in HST images of the Homunculus (Morse et al. 1998; Smith et al. 2000, 2004). This emission is seen in excess of violet starlight scattered by dust, and the strength of the excess increases into the far UV (Smith et al. 2004; hereafter Paper I)."[78]
Blues
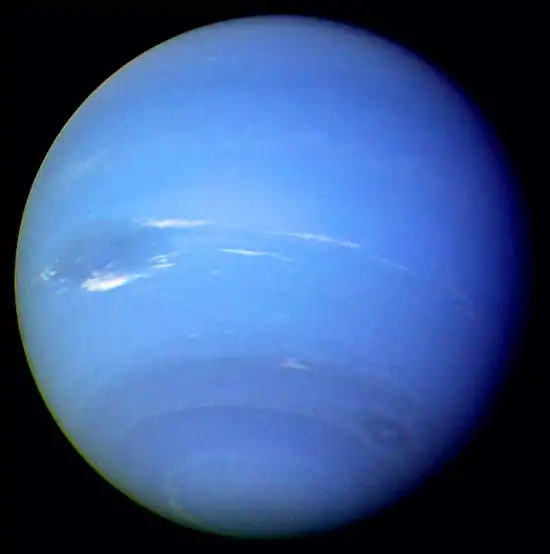
Blue is the wavelength range 450-475 nm.
A trace amount of methane is also present. Prominent absorption bands of methane occur at wavelengths above 600 nm, in the red and infrared portion of the spectrum. As with Uranus, this absorption of red light by the atmospheric methane is part of what gives Neptune its blue hue,[79] although Neptune's vivid azure differs from Uranus's milder cyan. Since Neptune's atmospheric methane content is similar to that of Uranus, some unknown atmospheric constituent is thought to contribute to Neptune's colour.[80]
Stars are often referred to by their predominant color. For example, blue stragglers are found among the galactic halo globular clusters.[81] Blue main sequence stars that are metal poor ([Fe/H] ≤ -1.0) are most likely not analogous to blue stragglers.[81]
"The Algol-type binaries are close, semi-detached, interacting binary star systems which contain a cool F–K giant or sub-giant secondary star that fills its Roche lobe and is losing mass to a hot B–A main sequence primary star."[82]
"The Algols contain a hot blue dwarf star with a magnetically-active late-type companion. In the close Algols, the gas stream flows directly into the photosphere of the blue mass-gaining star because it does not have enough room to avoid impact with that star."[82]
"[G]round-based UV [and blue astronomy] is a powerful facility for [the] study of [the] chemical evolution of [the] early Galaxy."[83] The UV and B astronomy are over the wavelength range 355.0-500.0 nm.[83]
Cyans
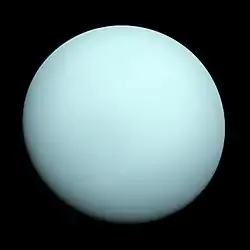
Cyan astronomy focuses on the wavelength range 476-495 nm.
Electric blue is a color close to cyan that is a representation of the color of lightning, an electric spark, and argon signs.
The electric blue glow of electricity results from the spectral emission of the excited ionized atoms (or excited molecules) of air (mostly oxygen and nitrogen) falling back to unexcited states, which happens to produce an abundance of electric blue light. This is the reason electrical sparks in air, including lightning, appear electric blue. It is a coincidence that the color of Cherenkov radiation and light emitted by ionized air are a very similar blue despite their very different methods of production.
Both Luna 24 and Apollo 12 soil samples are from mare soils that reflect primarily cyan that is likely due to the presence of TiO2 in the soils.[84]
"Previous work has suggested that a cyan color in the multispectral frame represents highland material, and that yellows and greens are freshly excavated basalts. However, we have recently found that a cyan color can also result from a freshly excavated high-Ti basalt."[85]
In larger amateur telescopes with an objective diameter of between 15 and 23 cm, the planet appears as a pale cyan disk with distinct limb darkening.
Methane possesses prominent absorption bands in the visible and near-infrared (IR) making Uranus aquamarine or cyan in color.[86]
Greens
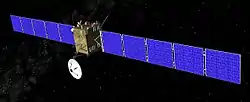
Green objects or emission lines in the green portion of the visible spectrum are the subject of green astronomy.

"Carroll and McCormack (1972) in Dublin reported complex spectra in the blue and green wavelength regions of both FeH and FeD".[87]
For elongated dust particles in cometary comas an investigation is performed at 535.0 nm (green) and 627.4 nm (red) peak transmission wavelengths of the Rosetta spacecraft's OSIRIS Wide Angle Camera broadband green and red filters, respectively.[88] "In the green, the polarization of the pure silicate composition qualitatively appears a better fit to the shape of the observed polarization curves".[88] "[B]ut they are characterized by a high albedo."[88] The silicates used to model the cometary coma dust are olivene (Mg-rich is green) and the pyroxene, enstatite.[88]
In December 2006, seven papers were published in the scientific journal, Science, discussing initial details of the sample analysis. Among the findings are: a wide range of organic compounds, including two that contain biologically usable nitrogen; indigenous aliphatic hydrocarbons with longer chain lengths than those observed in the diffuse interstellar medium; abundant amorphous silicates in addition to crystalline silicates such as olivine and pyroxene, proving consistency with the mixing of solar system and interstellar matter, previously deduced spectroscopically from ground observations;[89] hydrous silicates and carbonate minerals were found to be absent, suggesting a lack of aqueous processing of the cometary dust; limited pure carbon (CHON) was also found in the samples returned; methylamine and ethylamine was found in the aerogel but was not associated with specific particles. In the image at right the iron (Fe XIV) green line is followed by doppler imaging to show associated relative coronal plasma velocity towards (-7 km/s side) and away from (+7 km/s side) the large angle spectrometric coronagraph LASCO satellite camera.
Yellows
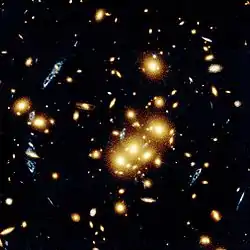
There are yellow objects and emission lines in the yellow portion of the visible spectrum to introduce yellow astronomy.
During the limb flares of December 18, 1956, a coronal line at 569.4 nm, a yellow line, occurred at 1822 UTC, 1900 UTC, undiminished up to 20,000 km above the solar limb, and at 2226 UTC, is identified as Ca XV.[90] "The coronal temperature was 4000000°."[90] "The December 18, 1956, flare appears to have been a violent condensation of material from a dense coronal cloud above an active region."[90]
The image at right shows several blue, loop-shaped objects that are multiple images of the same galaxy, duplicated by the gravitational lens effect of the cluster of yellow galaxies near the middle of the photograph. The lens is produced by the cluster's gravitational field that bends light to magnify and distort the image of a more distant object.
Oranges
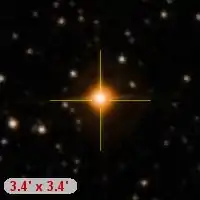
“Orange light at the wavelength of 590 nm is composed of photons having energy of 2.0 eV.”[91]
The Spite plateau (or Spite lithium plateau) is a baseline in the abundance of lithium found in old stars orbiting the galactic halo.
The curve on a graph of the abundance of lithium versus effective surface temperature formed a plateau among old halo stars for effective temperatures below about:
- log Teff ~ 3.75
or roughly 5,600 K. This suggested that the plateau represented the primordial abundance level of lithium in the Milky Way ... [with an estimate] that the abundance of lithium at the beginning of the galaxy was:
- NLi = (11.2 ± 3.8) × 10−11 NH
where NH is the abundance of hydrogen.[92]
"Lithium depletion through atomic diffusion has been suggested as a solution to the discrepancy between the Spite plateau abundance and the predicted value of the primordial lithium abundance"[93].
The orange system [in orange astronomy] is a number of emission lines very close together forming a band in the orange portion of the visible spectrum. These lines are usually associated with particular molecular species, including ScO, YO, and TiO.[94]
The variability of BD +50 961 (SY Persei, an orange star) is confirmed.[95]
Reds
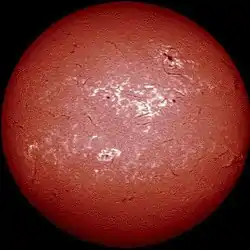
With respect to the color 'red', there are studies of the 'red shift' and the redness of objects such as the red dwarf AZ Cancri shown in the visual image at right. Red is 620-750 nm.
"Ideally all intrinsic colours should be found from unreddened stars. This is possible for dwarf and giant stars later than about A0 (Johnson, 1964) ... However, it cannot be used for stars of other spectral classes since they are all relatively infrequent in space, and generally reddened."[96]
Red supergiants (RSGs) are supergiant stars (luminosity class I) of spectral type K or M. They are the largest stars in the universe in terms of volume, although they are not the most massive. Betelgeuse and Antares are the best known examples of a red supergiant. These stars have very cool surface temperatures (3500–4500 K), and enormous radii. The five largest known red supergiants in the Galaxy are VY Canis Majoris, VV Cephei A, V354 Cephei, RW Cephei and KW Sagittarii, which all have radii about 1500 times that of the Sun (about 7 astronomical units, or 7 times as far as the Earth is from the Sun). The radius of most red giants is between 200 and 800 times that of the Sun. Absolute luminosities may reach -10 magnitude compared to +5 for our Sun.
Hypotheses
- Neptune was visible to visual astronomers within the last 40,000 years.
See also
- Alignment telescope
- Alternative ways to become an observer
- Babylonian astronomy
- Backyard Astronomy
- Chinese astronomy
- Dominant group/Astronomy
- Indian astronomy
- Islamic astronomy
- Light and optics
- Mathematical astronomy
- Pretelescopic astronomy
- Principles of Radiation Astronomy
- Skygazing
- Solar System
- Solar System and Stellar Astronomy
- Stargazing
- Theoretical astronomy
- Theoretical radiation astronomy
- The visible sky
References
- 1 2 Antony Cooke (2005). Visual Astronomy Under Dark Skies: A New Approach to Observing Deep Space. London: Springer-Verlag. p. 180. ISBN 1852339012. Retrieved 2011-11-06.
- ↑ Shorter Oxford English Dictionary, 5th Edition (2002)
- ↑ See Shorter Oxford English Dictionary, 5th Edition (2002); "of the colour of fresh milk or snow." See also Webster's New World Dictionary of American English, Third College Edition, (1988): "Having the color of pure snow or milk." See also The Random House College Dictionary of the English Language, Revised Edition,(1980)
- ↑ Shorter Oxford English Dictionary, 5th Edition (2002)
- ↑ Petit Larousse (2005). Missing or empty
|title=
(help) - ↑ Principles Of Instrumental Analysis F.James Holler, Douglas A. Skoog & Stanley R. Crouch 2006
- ↑ Tom Harris. How Fluorescent Lamps Work. Discovery Communications. Retrieved 27 June 2010.
- ↑ Philip B. Gove, ed. (1963). Webster's Seventh New Collegiate Dictionary. Springfield, Massachusetts: G. & C. Merriam Company. p. 1221.
- ↑ John E. Bortle (February 2001). The Bortle Dark-Sky Scale. Sky & Telescope. Retrieved 2009-11-18.
- ↑ Zezong, Xi, "The Discovery of Jupiter's Satellite Made by Gan De 2000 years Before Galileo", Chinese Physics 2 (3) (1982): 664–67.
- ↑ M. E. Lefébure (November 1900). "The Zodiacal Light according to the Ancients". The Observatory, A Monthly Review of Astronomy 23 (298): 393-8. http://adsabs.harvard.edu/cgi-bin/nph-data_query?bibcode=1900Obs....23..393.&link_type=ARTICLE&db_key=AST&high=. Retrieved 2011-11-08.
- 1 2 Shorter Oxford English Dictionary. 5th Edition (2002)
- 1 2 See also Webster's New World Dictionary of the American Language (1964)
- 1 2 Le Petit Larousse Illustré (1997)
- ↑ Webster's New World Dictionary of the American Language, Third College Edition.
- ↑ Shorter Oxford English Dictionary, 5th edition, 2002.
- ↑ Albedo - from Eric Weisstein's World of Physics. Scienceworld.wolfram.com. Retrieved 2011-08-19.
- ↑ P. R. Goode, et al. (2001). "Earthshine Observations of the Earth's Reflectance". Geophysical Research Letters 28 (9): 1671–4. doi:10.1029/2000GL012580. http://www.agu.org/journals/ABS/2001/2000GL012580.shtml.
- ↑ German Loan Words in English. German.about.com (2010-06-22). Retrieved on 2011-06-05.
- ↑ Essentials of Geology, 3rd Edition, Stephen Marshak, p 182
- ↑ Darryl Powell. Quartzite. Mineral Information Institute. Retrieved 2009-09-09.
- ↑ Deer, W.A.; Howie, R.A., & Zussman, J. (1992). An introduction to the rock forming minerals (2nd edition ed.). London: Longman ISBN 0-582-30094-0
- ↑ Hurlbut, Cornelius S.; Klein, Cornelis (1985). Manual of Mineralogy, Wiley, (20th edition ed.). ISBN 0-471-80580-7
- ↑ W. A. H. Rushton (1 June 1966). "Densitometry of pigments in rods and cones of normal and color defective subjects" (PDF). Investigative Ophthalmology 5 (3): 233–41. PMID 5296487. http://www.iovs.org/cgi/content/abstract/5/3/233. Retrieved 2006-11-14.
- ↑ Martin V. Zombeck (1990). Calibration of MK spectral types, In: Handbook of Space Astronomy and Astrophysics (2nd ed.). Cambridge University Press. p. 105. ISBN 0-521-34787-4.
- ↑ David F. Gray (November 1992). "The Inferred Color Index of the Sun". Publications of the Astronomical Society of the Pacific 104 (681): 1035-8.
- ↑ Rigel.
- ↑ A. Léger; L. d'Hendecourt (May 1985). "Are polycyclic aromatic hydrocarbons the carriers of the diffuse interstellar bands in the visible?". Astronomy and Astrophysics 146 (1): 81-5.
- ↑ Cecie Starr (2005). Biology: Concepts and Applications. Thomson Brooks/Cole. ISBN 053446226X.
- ↑ CIE (the International Commission on Illumination)
- ↑ CIE International Lighting Vocabulary
- ↑ Palmer and Grant, The Art of Radiometry
- ↑ M. O. Roman; C.B. Schaaf; P. Lewis; F. Gao; G.P. Anderson; J.L. Privette; A.H. Strahler; C.E. Woodcock et al. (2010). "Assessing the Coupling between Surface Albedo derived from MODIS and the Fraction of Diffuse Skylight over Spatially-Characterized Landscapes". Remote Sensing of Environment 114 (4): 738–60. doi:10.1016/j.rse.2009.11.014.
- ↑ Dan Bruton. Conversion of Absolute Magnitude to Diameter for Minor Planets. Department of Physics & Astronomy (Stephen F. Austin State University). Retrieved 2008-10-07.
- ↑ Pridmore, R. “Effect of purity on hue (Abney effect) in various conditions.” Color Research and Application. 32.1 (2007): 25–39.
- ↑ The Perception of Color
- ↑ Glenn Kennel (2006). Color and Mastering for Digital Cinema. Focal Press. ISBN 0-240-80874-6.
- 1 2 Morrison P (1967). "Extrasolar X-ray Sources". Ann Rev Astron Astrophysics 5 (1): 325. doi:10.1146/annurev.aa.05.090167.001545.
- ↑ Dictionary.com. The American Heritage Dictionary of the English Language, Fourth Edition. Houghton Mifflin Company, 2004. (accessed: January 25, 2008).
- ↑ W.J. Jones and B.P. Stoicheff, ‘Inverse Raman Spectra: Induced Absorption at Optical Frequencies’, Phys. Rev. Lett. 13, 657 - 9 (1964).
- 1 2 3 David Merritt (2013). Dynamics and Evolution of Galactic Nuclei. Princeton, NJ: Princeton University Press. ISBN 9781400846122.
- ↑ B.M. Peterson & K. Horne Reverberation Mapping of Active Galactic Nuclei (2004)
- ↑ G. D. Mahan (April 24, 1972). "Violet satellite bands in the spectra of Li perturbed by He". Physics Letters A 39 (2): 145-6. doi:10.1016/0375-9601(72)91056-0. http://www.sciencedirect.com/science/article/pii/0375960172910560. Retrieved 2013-03-23.
- ↑ L. Monaco; S. Villanova; C. Moni Bidin; G. Carraro; D. Geisler; P. Bonifacio; O. A. Gonzalez; M. Zoccali et al. (May 2011). "Lithium-rich giants in the Galactic thick disk". Astronomy & Astrophysics 529 (5): 10. doi:10.1051/0004-6361/201016285.
- 1 2 Harvey B. Richer (February 1981). "Observations of a complete sample of carbon stars in the Large Magellanic Cloud". The Astrophysical Journal 243 (2): 744-55. doi:10.1086/158642.
- ↑ D. R. Bates (October 1978). "Forbidden oxygen and nitrogen lines in the nightglow". Planetary and Space Science 26 (10): 897-912. doi:10.1016/0032-0633(78)90073-9. http://www.sciencedirect.com/science/article/pii/0032063378900739. Retrieved 2013-01-16.
- ↑ R. J. Thomas; R. A. Young (January 1981). "Measurement of atomic oxygen and related airglows in the lower thermosphere". Journal of Geophysical Research: Oceans 86 (08): 7389-93. doi:10.1029/JC086iC08p07389. http://onlinelibrary.wiley.com/doi/10.1029/JC086iC08p07389/abstract. Retrieved 2013-01-16.
- 1 2 3 K. J. McCarthy; A. Baciero; B. Zurro; TJ-II Team (June 12, 2000). Impurity Behaviour Studies in the TJ-II Stellarator, In: 27th EPS Conference on Contr. Fusion and Plasma Phys. (PDF). 24B. Budapest: ECA. pp. 1244–7. Retrieved 2013-01-20.
- ↑ Harold D. Babcock (May 1923). "A study of the green auroral line by the interference method". The Astrophysical Journal 57 (05): 209-21. doi:10.1086/142747. http://adsabs.harvard.edu/full/1923ApJ....57..209B. Retrieved 2013-01-18.
- ↑ Yoichi Takeda; Eiji Kambe; Kozo Sadakane; Seiji Masuda (October 2010). "Oxygen and Neon Abundances of B-Type Stars in Comparison with the Sun". Publications of the Astronomical Society of Japan 65 (5): 1239-48. http://arxiv.org/pdf/1008.1220.pdf. Retrieved 2013-01-18.
- ↑ Miller, J.F., Evans, K.R., Rovey, C.W., II, Ausich, W.L., Bolyard, S.E., Davis, G.H., Ethington, R.L., Sandberg, C.A., Thompson, T.L., and Waters, J.A., Mixed-age echinoderms, conodonts, and other fossils used to date a meteorite impact, and implications for missing strata in the type Osagean (Mississippian) in Missouri, USA. Echinoderm Paleobiology, 2008, 53p.
- ↑ David Rajmon (2009-07-01). Impact database 2009.1. Retrieved 2009-08-25.
- ↑ Beveridge, T.R., 1949, The Geology of the Weaubleau quadrangle, Missouri [Ph.D. thesis]: Iowa City, State University of Iowa
- ↑ Snyder, F.G., Gerdemann, P.E., Hendricks, H.E., Williams, J.H., Wallace, G., and Martin, J.A., 1965, Cryptoexplosive structures in Missouri: Guidebook, 1965 Annual Meeting of the Geological Society of America: Missouri Geological Survey and Water Resources, Report of Investigations No. 30, 73 p.
- 1 2 Evans, Kevin R.; Mickus, Kevin L.; Rovey, Charles W. III; & Davis, George H. (2003). Field Trip I: The Weaubleau Structure: Evidence of a Mississippian Meteorite Impact in Southwestern Missouri. Association of Missouri Geologists Field Trip Guidebook, No. 26, 50th Annual Meeting, pp. 1-30. Missouri Department of Natural Resources. PDF
- ↑ MeteorObs Explanations and Definitions (states IAU definition of a fireball). Meteorobs.org. 1999-07-09. Retrieved 2011-09-16.
- ↑ International Meteor Organization - Fireball Observations. Imo.net. 2004-10-12. Retrieved 2011-09-16.
- ↑ Fireball Report: 4589 records found between 2011-01-01 and 2011-12-31. American Meteor Society. Retrieved 2012-04-24.
- ↑ MJS Belton (2004). Mitigation of hazardous comets and asteroids. Cambridge University Press. ISBN 0-521-82764-7.:156
- ↑ Petrus M. Jenniskens (October 20, 2012). 2012, October 20 - FIRST METEORITE FOUND!. San Francisco, California: NASA Ames Research Center. Retrieved 2012-10-22.
- ↑ Krasnopolsky, V. A.; Parshev, V. A. (1981). "Chemical composition of the atmosphere of Venus". Nature 292 (5824): 610–613. doi:10.1038/292610a0.
- ↑ Vladimir A. Krasnopolsky (2006). "Chemical composition of Venus atmosphere and clouds: Some unsolved problems". Planetary and Space Science 54 (13–14): 1352–1359. doi:10.1016/j.pss.2006.04.019.
- ↑ Environmental Encyclopedia, 3rd ed., Thompson Gale, 2003, ISBN 0-7876-5486-8
- 1 2 Jay Winston (1971). "The Annual Course of Zonal Mean Albedo as Derived From ESSA 3 and 5 Digitized Picture Data". Monthly Weather Review 99(11) (11): 818–27. doi:10.1175/1520-0493(1971)099<0818:TACOZM>2.3.CO;2.
- ↑ The Climate System. Manchester Metropolitan University. Retrieved 2007-11-11.
- ↑ Richard A. Betts (2000). "Offset of the potential carbon sink from boreal forestation by decreases in surface albedo". Nature 408 (6809): 187–90. doi:10.1038/35041545. PMID 11089969.
- ↑ "Changing Greenland - Melt Zone" page 3, of 4, article by Mark Jenkins in National Geographic June 2010, accessed July 8, 2010
- ↑ http://vih.freeshell.org/pp/01-ONW-St.Petersburg/Fresnel.pdf
- ↑ Baffled Scientists Say Less Sunlight Reaching Earth. LiveScience. 2006-01-24. Retrieved 2011-08-19.
- ↑ Jeffrey S. Medkeff (2002). Lunar Albedo. Retrieved 5 July 2010.
- ↑ Sue Lavoie (April 29, 2000). PIA02393: South Polar Cap, Summer 2000. Pasadena, California USA: NASA/JPL. Retrieved 2013-05-01.
- 1 2 3 Tony Greicius (January 12, 2012). Brilliant Enceladus. Washington, D.C.: NASA. Retrieved 2012-08-09.
- ↑ Robert Roy Britt (2001-11-29). Comet Borrelly Puzzle: Darkest Object in the Solar System. Space.com. Retrieved 2012-09-01.
- ↑ Tony Hoffman. Comet West: The Great Comet of 1976. Earthlink. Retrieved 2013-05-02.
- ↑ B. Sicardy; J. L. Ortiz; M. Assafin; E. Jehin; A. Maury; E. Lellouch; R. Gil-Hutton; F. Braga-Ribas et al. (2011). "Size, density, albedo and atmosphere limit of dwarf planet Eris from a stellar occultation". European Planetary Science Congress Abstracts 6: 137. http://meetingorganizer.copernicus.org/EPSC-DPS2011/EPSC-DPS2011-137-8.pdf. Retrieved 2011-09-14.
- ↑ W. H. Wright (August 1927). "Photographs of Venus made by Infra-red and by Violet Light". Publications of the Astronomical Society of the Pacific 39 (230): 220-1. doi:10.1086/123718.
- ↑ P.C. Thomas; J.A. Burns; L. Rossier; D. Simonelli; J. Veverka; C.R. Chapman; K. Klaasen; T.V. Johnson et al. (September 1998). "The Small Inner Satellites of Jupiter". Icarus 135 (1): 360-71. doi:10.1006/icar.1998.5976. http://www.sciencedirect.com/science/article/pii/S0019103598959760. Retrieved 2012-06-01.
- ↑ Nathan Smith; Jon A. Morse; Nicholas R. Collins; Theodore R. Gull (August 2004). "The Purple Haze of η Carinae: Binary-induced Variability?". The Astrophysical Journal 610 (2): L105-8. doi:10.1086/423341.
- ↑ D. Crisp; H. B. Hammel (June 14, 1995). Hubble Space Telescope Observations of Neptune. Hubble News Center. Retrieved April 22, 2007.
- ↑ Kirk Munsell; Harman Smith; Samantha Harvey (November 13, 2007). Neptune overview, In: Solar System Exploration. NASA. Retrieved February 20, 2008.
- 1 2 Preston, G. W.; Beers, T. C.; Shectman, S. A. (December 1993). "The Space Density and Kinematics of Metal-Poor Blue Main Sequence Stars Near the Solar Circle". Bulletin of the American Astronomical Society 25 (12): 1415.
- 1 2 M.T. Richards (March 2004). "Doppler tomography of Algols". Astronomische Nachrichten 325 (3): 229-32. doi:10.1002/asna.200310206. http://www2.astro.psu.edu/users/charlton/Astro20/richards.pdf. Retrieved 2012-03-05.
- 1 2 Klochkova, Valentina; Ermakov, Sergey; Panchuk, Vladimir; Zhao, Gang (July 2007). Ana I. Gómez de Castro and Martin A. Barstow (ed.). High resolution spectroscopy of halo stars within the spectral region 3550-5000 °A°A, In: UV Astronomy: Stars from Birth to Death. Proceedings of the Joint Discussion n.4 during the IAU general Assembly of 2006. International Astronomical Union. p. 161. Bibcode:2007uasb.conf..161K. ISBN 978-84-7491-852-6.
- ↑ Carle’ M. Pieters. Mare basalt types on the front side of the moon - A summary of spectral reflectance data, In: Lunar and Planetary Science Conference, 9th, Houston, Tex., March 13-17, 1978, Proceedings. 3. New York: Pergamon Press, Inc. pp. 2825–49. Bibcode:1978LPSC....9.2825P.
- ↑ D. J. Heather; S. K. Dunkin; P. D. Spudis; D. B. J. Bussey (January 1999). A Multispectral Analysis of the Flamsteed Region of Oceanus Procellarum, In: Workshop on New Views of the Moon 2: Understanding the Moon Through the Integration of Diverse Datasets. Bibcode:1999nvm..conf...24H.
- ↑ Jonathan I. Lunine (1993). "The Atmospheres of Uranus and Neptune". Annual Review of Astronomy and Astrophysics 31: 217–263. doi:10.1146/annurev.aa.31.090193.001245.
- ↑ John G. Phillips; Sumner P. Davis; Bo Lindgren; Walter J. Balfour (December 1987). "The near-infrared spectrum of the FeH molecule". The Astrophysical Journal Supplement Series 65 (12): 721-78. doi:10.1086/191241.
- 1 2 3 4 I. Bertini; N. Thomas; C. Barbieri (January 2007). "Modeling of the light scattering properties of cometary dust using fractal aggregates". Astronomy & Astrophysics 461 (1): 351-64. doi:10.1051/0004-6361:20065461. http://www.aanda.org/articles/aa/pdf/2007/01/aa5461-06.pdf. Retrieved 2011-12-08.
- ↑ The building blocks of planets within the 'terrestrial' region of protoplanetary disks. nottingham.ac.uk. Retrieved 2008-03-04.
- 1 2 3 Harold Zirin (March 1959). "Physical Conditions in Limb Flares and Active Prominences. II. a Remarkable Limb Flare, December 18, 1956". Astrophysical Journal 129 (3): 414-23. doi:10.1086/146633.
- ↑ S. Kulmala, J. Suomi (2003). "Current status of modern analytical luminescence methods". Analytica Chimica Acta 500: 21-69. doi:10.1016/j.aca.2003.09.004. http://nathan.instras.com/documentDB/paper-362.pdf. Retrieved 2012-01-07.
- ↑ F. Spite, M. Spite (November 1982). "Abundance of lithium in unevolved halo stars and old disk stars - Interpretation and consequences". Astronomy and Astrophysics 115 (2): 357–366.
- ↑ K. Lind; M. Asplund; P. S. Barklem (August 2009). "Departures from LTE for neutral Li in late-type stars". Astronomy and Astrophysics 503 (2): 541-4. doi:10.1051/0004-6361/200912221.
- ↑ G. H. Herbig (March 1974). "VY Canis Majoris. IV. The emission bands of ScO". The Astrophysical Journal 188 (3): 533-8. doi:10.1086/152744.
- ↑ T. W. Backhouse (July 1899). "Confirmed or New Variable Stars". The Observatory 22 (281): 275-6.
- ↑ M. Pim FitzGerald (February 1970). "The Intrinsic Colours of Stars and Two-Colour Reddening Lines". Astronomy and Astrophysics 4 (2): 234-43.
Further reading
- Tenorio-Tagle G, Bodenheimer P (1988). "Large-scale expanding superstructures in galaxies". Annual Review of Astronomy and Astrophysics 26: 145–97. http://articles.adsabs.harvard.edu/cgi-bin/nph-iarticle_query?1988ARA%26A..26..145T.
External links
- Bing Advanced search
- Google Books
- Google scholar Advanced Scholar Search
- International Astronomical Union
- JSTOR
- Lycos search
- NASA/IPAC Extragalactic Database - NED
- NASA's National Space Science Data Center
- NCBI All Databases Search
- Office of Scientific & Technical Information
- PubChem Public Chemical Database
- Questia - The Online Library of Books and Journals
- SAGE journals online
- The SAO/NASA Astrophysics Data System
- Scirus for scientific information only advanced search
- SDSS Quick Look tool: SkyServer
- SIMBAD Astronomical Database
- Spacecraft Query at NASA
- SpringerLink
- Taylor & Francis Online
- Universal coordinate converter
- Wiley Online Library Advanced Search
- Yahoo Advanced Web Search
{{Radiation astronomy resources}}