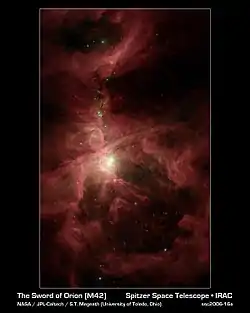
Def. a suspension of dry dust in an atmosphere is called a lithometeor.
"A lithometeor consists of solid particles suspended in the air or lifted by the wind from the ground."[1]
"A lithometeor is the general term for particles suspended in a dry atmosphere; these include dry haze, smoke, dust, and sand."[2]
"Dry haze is an accumulation of very fine dust or salt particles in the atmosphere; it does not block light, but instead causes light rays to scatter. Dry haze particles produce a bluish color when viewed against a dark background, but look yellowish when viewed against a lighter background. This light-scattering phenomenon (called Mie scattering) also causes the visual ranges within a uniformly dense layer of haze to vary depending on whether the observer is looking into the sun or away from it."[2]
Heavy metal pollution may occur in lithometeors.[3]
"The rise of airborne dust is constantly augmenting from the desert (Bilma) to the southern Sahelian stations (Niamey) where it has increased by a factor five. ... the Sahelian zone with airborne dust during the 80s ... All stations have recorded a general increase of wind velocity. The increase of lithometeors frequency as well as the wind velocity during the drought period is not explained by the aridification."[4]
Minerals
"Optical constants of natural minerals are of interest for characterizing interstellar dust, for remote sensing of terrain and for light scattering in the atmosphere by soil particles."[5]
Emissions
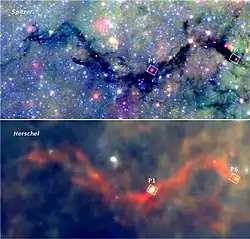
The "discovery of the anomalous dust-correlated microwave emission (AME) in the galaxy [was] by Leitch et al (1997) [18] [Characteristics include]
- the AME constitutes a foreground emission to cosmic microwave background (CMB) radiation. [...]
- it provides a window into the properties of small grains, which play crucial roles for the physics and chemistry of the ISM.
- [It is a] diffuse and localized AME"[6]
"In the case of electric dipole radiation, the associated fluctuation in angular momentum is due to absorption of and decays stimulated by microwave photons (dominated by Cosmic Microwave Background (CMB) photons in the diffuse ISM)."[6]
"The [warm ionized medium] WIM is characterized by a large gas temperature T ≈ 8000 K, and a fully ionized gas at low density, nH+ ≈ 0.1 cm-3. Collisions with ions provide the dominant excitation mechanism. Grains are mostly negatively charged due to the high rate of sticking collisions with high-velocity electrons. For a coronene molecule, the characteristic time between ion collisions and the characterstic rotational damping time at the peak angular momentum τrot = √ττed turn out to be comparable6, of order a few years."[6]
The "peak emissivity is enhanced by about 23% for the WIM [and only 11 % for the warm neutral medium (WNM)], although the peak frequency remains unchanged."[6]
"A more important effect on the spectrum is that of increasing the characteristic internal temperature Tω, which makes the grains wobble rather than simply spin about their axis of greatest inertia."[6]
For triaxiality there is an "additional enhancement of the peak frequency and total power by up to the same factors (~ 30 % and 2, respectively) for a large internal relaxation temperature and highly elliptical grains."[6]
"The spectrum includes dust continuum, molecular rotation line and atomic fine-structure line emissions."[7]
"Stretching across almost 100 light-years of space, the Snake nebula is located about 11,700 light-years from Earth in the direction of the constellation Ophiuchus."[8]
"In images from NASA's Spitzer Space Telescope, which observes infrared light, it appears as a sinuous, dark tendril against the starry background. It was targeted because it shows the potential to form many massive stars (stars with more than 8 times the mass of our Sun). SMA was used to observe sub-millimetre radiation from the nebula, radiation emitted between the infrared and radio parts of the electromagnetic spectrum."[8]
"The two panels [at right] show the Snake nebula as photographed by the Spitzer and Herschel space telescopes. At mid-infrared wavelengths (the upper panel taken by Spitzer), the thick nebular material blocks light from more distant stars. At far-infrared wavelengths, however (the lower panel taken by Herschel), the nebula glows due to emission from cold dust. The two boxed regions, P1 and P6, were examined in more detail by the Submillimeter Array."[8]
"To learn how stars form, we have to catch them in their earliest phases, while they're still deeply embedded in clouds of gas and dust, and the SMA is an excellent telescope to do so."[9]
"The team studied two specific spots within the Snake nebula, designated P1 and P6. Within those two regions they detected a total of 23 cosmic "seeds" -- faintly glowing spots that will eventually give birth to between one and a few stars. The seeds generally weigh between 5 and 25 times the mass of the Sun, and each spans a few hundred billion kilometres (for comparison the average Earth-Sun distance is 150 million km). The sensitive, high-resolution SMA images not only unveil the small seeds, but also differentiate them in age."[8]
"Previous theories proposed that high-mass stars form within very massive, isolated "cores" weighing at least 100 times the mass of the Sun. These new results show that that is not the case. The data also demonstrate that massive stars aren't born alone but in groups."[8]
Backgrounds
"The differential 850-μm counts are well described by the function
where is the flux in mJy, = 3.0 × 104 per square degree per mJy, and = 0.4 − 1.0 is chosen to match the 850-μm extragalactic background light."[10]
The "absorption and reradiation of light by dust in the history of galaxy formation and evolution is [...] the submillimeter extragalactic background light [(EBL). It] has approximately the same integrated energy density as the optical EBL."[10]
Meteors

"[V]isible meteors consist of 0.1- to 1-mm-sized debris from active comets (Williams 1990)."[11]
"This dramatic new image of cosmic clouds in the constellation of Orion reveals what seems to be a fiery ribbon in the sky. The orange glow represents faint light coming from grains of cold interstellar dust, at wavelengths too long for human eyes to see. It was observed by the ESO-operated Atacama Pathfinder Experiment (APEX) in Chile."[12]
"In this image, the submillimetre-wavelength glow of the dust clouds is overlaid on a view of the region in the more familiar visible light, from the Digitized Sky Survey 2. The large bright cloud in the upper right of the image is the well-known Orion Nebula, also called Messier 42."[12]
Electrons
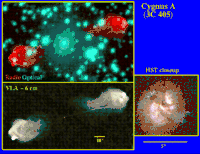
"Many objects with jets, especially the powerful FR II radio sources with long and highly collimated jets, show hot spots - compact enhancements in brightness of the lobes. Cygnus A [at right] is a prime example. These may in turn have internal structure, and often have the flattest spectra (thus most energetic particle populations) in the extended lobes. They have been pictures as encounter surfaces between the jet flows and a mostly unseen surrounding medium, with compression of the magnetic field occurring and thus vastly increased emissivity. Some (such as Pictor A) have such high-energy electron populations that sychrotron emission continues through the optical into the X-ray regime."[13]
Cygnus A "is the most powerful radio galaxy on our corner of the Universe, used as a point of departure for studying radio galaxies at great distances. At a redshift z=0.0565 (distance of about 211 Mpc or 700 million light-years), its nature remains mysterious enough. The first photographs of Cygnus A showed two clumps of luminous material, which led [to the speculation] that the radio emission was somehow linked to a galaxy collision. [Or,] a poorly resolved version of Centaurus A, bisected by a thick dust lane. The HST image shown as an inset [in the image at right] reveals much detail, but doesn't quite clear the matter up. We see dust and an odd Z-shaped pattern. Much of this light in some regions comes not from stars, but from gas ionized by the nucleus. This is a narrow-line radio galaxy, but infrared and polarization measurements show that from some directions it would appear as a broad-line object and perhaps as a quasar, so that there is plenty of radiation in some directions to light up the gas."[14]
"Cygnus A is an excellent example of the Fanaroff-Riley (FR) type II radio sources, characterized by faint, very narrow jets, distinct lobes, and clear hot spots at the outer edges of the lobes, often where the jets intersect the outer edges. These are in general more powerful radio sources than the FR I objects [...], with the difference being frequently attributed to faster (relativistic?) motion of the jet material in the stronger FR II sources. The radio/optical overlay highlights the extent of the radio source beyond the central galaxy, extending 140 kpc (500,000 light-years) if we see it sideways."[14]
Gamma rays
A "fading counterpart to GRB 980329 at 850 μm [has been found]. [...] the sub-millimeter flux was relatively bright. [...] The radio through sub-millimeter spectrum of GRB 980329 is well fit by a power law with index α = +0.9. However, we cannot exclude a ν1/3 power law attenuated by synchrotron self-absorption."[15]
Submillimeter observations
- determine "the breaks in the radio to sub-millimeter to optical spectrum so that the spectral shape can be compared to the synchrotron models"[15]
- determine "the evolution of the sub-millimeter flux"[15] and
- look "for underlying quiescent sources that may be dusty star-forming galaxies at high redshifts."[15]
X-rays
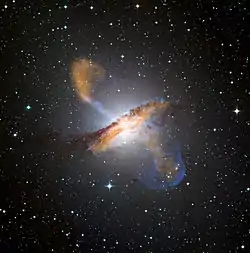
"The BeppoSAX GRB Monitor was triggered on 1998 March 29.156 UT (Frontera et al. 1998a) [GRB 980329]. This was the brightest burst that had been seen simultaneously by the BeppoSAX Wide Field Camera, with a peak flux ~ 6 Crab in the 2-26 keV band. A fading X-ray source 1SAX J0702.6+3850 was found using the BeppoSAX Narrow Field Instruments (in't Zand et al. 1998)."[15]
At right is "[c]olour composite image of Centaurus A [NGC 5128], revealing the lobes and jets emanating from the active galaxy’s central black hole. This is a composite of images obtained with three instruments, operating at very different wavelengths. The 870-micron submillimetre data, from LABOCA on APEX, are shown in orange. X-ray data from the Chandra X-ray Observatory are shown in blue. Visible light data from the Wide Field Imager (WFI) on the MPG/ESO 2.2 m telescope located at La Silla, Chile, show the stars and the galaxy’s characteristic dust lane in close to "true colour"."[16]
The optical blue band is centered at 445 nm, the optical green band is centered at 551 nm, and the optical red is centered at 658 nm. The submillimeter band (Atacama Pathfinder Experiment) in yellow is centered at 870 µm.
Opticals
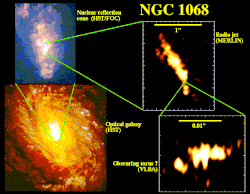
"Measurements of the polarization of the light near the nucleus of NGC 1068, a nearby and prototypical type 2 Seyfert, provided strong evidence that it actually contains a type 1 nucleus which is blocked from our direct view by an obscuring ring or torus of material. The nucleus produces a radio jet at right angles to this hypothesized torus, which must lie almost at right angles to the galaxy's disk plane. Recent VLBI observations may have detected this torus, as shown in this montage. HST images are used to show the galaxy as a whole and the conelike illumination pattern of highly ionized gas which must see the nucleus directly, then the radio jet and finally a tiny structure which has the right size, orientation, and temperature to be the obscuring disk. If this in fact the obscuring material, this is an important piece of evidence for the unified scheme for Seyfert galaxies. This is simply the notion that many type 2 Seyferts would be type 1 objects if we could see them from the proper direction, nearly along the axis of the torus so that our view is not blocked. These special directions are often marked by both radio jets and cones of intense radiation, which we see either as they ionize ambient gas or are reflected from clouds rich in dust that happen to lie within the cones."[17]
Visuals
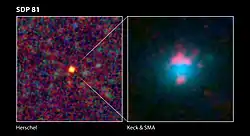
"This image composite shows a warped and magnified view of a galaxy discovered by the Herschel Space Observatory, one of five such galaxies uncovered by the infrared telescope. The galaxy -- referred to as "SDP 81" -- is the yellow dot in the left image taken by Herschel. It can also be seen as the pink smudges in the right image, a composite of ground-based observations showing more detail."[18]
"Herschel was able to find the galaxy, which is buried in dust, because it happens to be positioned behind another galaxy (blue blob at right), which is acting like a cosmic lens to make it appear brighter. The gravity of the foreground galaxy is distorting and magnifying the distant galaxy's light, causing it to appear in multiple places, as seen as the pink smudges. The distant galaxy is so far away that its light took about 11 billion years to reach us."[18]
"Herschel couldn't detect the foreground galaxy, but astronomers were able to spot it in visible light using the W.M. Keck Observatory. Several follow-up observations by ground telescopes helped to get a better view of the distant galaxy. For example, the pink smudges at the right show wavelengths that are even longer than what Herschel sees in the submillimeter portion of the electromagnetic spectrum. Those observations were made by the Smithsonian Astrophysical Observatory's Submillimeter Array in Hawaii."[18]
Infrareds
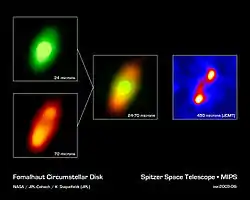
"It was not until after the variable radio source was discovered that infrared observations [of GRB 980329] found a fading counterpart (Klose et al. 1998; Palazzi et al. 1998; Metzger 1998): this indicated that the optical extinction was significant for this source (Larkin et al. 1998; Taylor et al. 1998b), and/or the redshift was large (Fruchter 1999)."[15]
"Starting on 1998 April 5, we made a series of photometry observations of VLA J070238.0+385044 using SCUBA. [...] On April 5.2 UT, we detected the source at 850 μm with a flux density of 5 ± 1.5 mJy. This source was confirmed on April 6.2 with a flux density of 4 ± 1.2 mJy, resulting in an average of 4.5 ± 1 mJy over the two days."[15]
"The NASA Spitzer Space Telescope has obtained the first infrared images of the dust disc surrounding Fomalhaut, the 18th brightest star in the sky. Planets are believed to form from such a flattened disc-like cloud of gas and dust orbiting a star very early in its life. The Spitzer telescope was designed in part to study these circumstellar discs, where the dust particles are so cold that they radiate primarily at infrared wavelengths. Located in the constellation Piscis Austrinus, the parent star and its putative planetary system are found at a distance of 25 light-years."[19]
"Twenty years ago, the Infrared Astronomical Satellite, the first orbiting infrared telescope, detected much more infrared radiation coming from Fomalhaut than was expected for a normal star of this type. The dust is presumed to be debris left over from the formation of a planetary system. However, the satellite did not have adequate spatial resolution to image the dust directly. Subsequent measurements with sub-millimeter radio telescopes suggested that Fomalhaut is surrounded by a huge dust ring 370 astronomical units (an astronomical unit is the average distance between the Sun and Earth), or 34 billion miles (56 billion kilometers) in diameter. This corresponds to a size of nearly five times larger than our own solar system. Moreover, the sub-millimeter observations (far right image) revealed that the ring was inclined 20 degrees from an edge-on view."[19]
"The new images obtained with the multiband imaging photometer onboard Spitzer confirm this general picture, while revealing important new details of Fomalhaut's circumstellar dust. The 70-micron data (red) clearly shows an asymmetry in the dust distribution, with the southern lobe one-third brighter than the northern. Such an unbalanced structure could be produced by a collision between moderate-sized asteroids in the recent past (releasing a localized cloud of dust) or by the steering effects of ring particles by the gravitational influence of an unseen planet."[19]
"At 24 microns (green), the Spitzer image shows that the center of the ring is not empty. [Note that an image of a reference star was subtracted from the Fomalhaut image to reveal the faint disc emission.] Instead, the 'doughnut hole' is filled with warmer dust that extends inward to within at least 10 astronomical units of the parent star. This warm inner disc of dust occupies the region that is most likely to be occupied by planets and may be analogous to our solar system's 'zodiacal cloud' -- but with considerably more dust. One possible explanation for this warmer dust is that comets are being nudged out of the circumstellar ring by the gravitational influence of massive planets. These comets then loop in toward the central star, releasing dust particles just as comets do in our own solar system."[19]
Submillimeters
“[T]erahertz radiation refers to electromagnetic waves propagating at frequencies in the terahertz range. It is synonymously termed submillimeter radiation, terahertz waves, terahertz light, T-rays, T-waves, T-light, T-lux, THz. The term typically applies to electromagnetic radiation with frequencies between high-frequency edge of the microwave band, 300 gigahertz (3 x 1011 Hz),"[20] and the long-wavelength edge of far-infrared light, 3000 GHz (3 x 1012 Hz or 3 THz). In wavelengths, this range corresponds to 0.1 mm (or 100 µm) infrared to 1.0 mm microwave.
Terahertz radiation is emitted as part of the black body radiation from anything with temperatures greater than about 10 kelvin. While this thermal emission is very weak, observations at these frequencies are important for characterizing the cold 10-20K dust in the interstellar medium in the Milky Way galaxy, and in distant starburst galaxies. Telescopes operating in this band include the James Clerk Maxwell Telescope, the Caltech Submillimeter Observatory and the Submillimeter Array at the Mauna Kea Observatory in Hawaii, the BLAST balloon borne telescope, the Herschel Space Observatory, and the Heinrich Hertz Submillimeter Telescope at the Mount Graham International Observatory in Arizona. The Atacama Large Millimeter Array, under construction, will operate in the submillimeter range. The opacity of the Earth's atmosphere to submillimeter radiation restricts these observatories to very high altitude sites, or to space.
Hydrogens
The hydrogen line, 21 centimeter line or HI line refers to the electromagnetic radiation spectral line that is created by a change in the energy state of neutral hydrogen atoms. This electromagnetic radiation is at the precise frequency of 1420.40575177 [megahertz] MHz, which is equivalent to the vacuum wavelength of 21.10611405413 cm in free space. This wavelength or frequency falls within the microwave radio region of the electromagnetic spectrum, and it is observed frequently in radio astronomy, since those radio waves can penetrate the large clouds of interstellar cosmic dust that are opaque to visible light.
Compounds
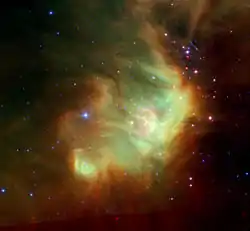
"Baby stars are forming near the eastern rim of the cosmic cloud Perseus, in this infrared image from NASA's Spitzer Space Telescope."[21]
"The baby stars are approximately three million years old and are shown as reddish-pink dots to the right of the image. The pinkish color indicates that these infant stars are still shrouded by the cosmic dust and gas that collapsed to form them. These stars are part of the IC348 star cluster, which consists of over 300 known member stars."[21]
"The Perseus Nebula can be seen as the large green cloud at the center of the image. Wisps of green are organic molecules called Polycyclic Aromatic Hydrocarbons (PAHs) that have been illuminated by the nearby star formation. Meanwhile, wisps of orange-red are dust particles warmed by the newly forming stars. The Perseus Nebula is located about 1,043 light-years away in the Perseus constellation."[21]
"The image is a three channel false color composite, where emission at 4.5 microns is blue, emission at 8.0 microns is green, and 24-micron emission is red."[21]
Alloys
"The broad, 60 < FWHM < 100 nm, featureless luminescence band known as extended red emission (ERE) is seen in such diverse dusty astrophysical environments as reflection nebulae17, planetary nebulae3, HII regions (Orion)12, a Nova11, Galactic cirrus14, a dark nebula7, Galaxies8,6 and the diffuse interstellar medium (ISM)4. The band is confined between 540-950 nm, but the wavelength of peak emission varies from environment to environment, even within a given object. ... the wavelength of peak emission is longer and the efficiency of the luminescence is lower, the harder and denser the illuminating radiation field is13. These general characteristics of ERE constrain the photoluminescence (PL) band and efficiency for laboratory analysis of dust analog materials."[22]
"The PL efficiencies measured for HAC and Si-HAC alloys are consistent with dust estimates for reflection nebulae and planetary nebulae, but exhibit substantial photoluminescence below 540 nm which is not observed in astrophysical environments."[22]
"Optical constants measured at normal incidence for iron (Bolotin et al., 1969) and for iron-nickel alloys (Sasovskaya and Noskov, 1974) also predict a red-sloped spectrum."[23]
Materials
.jpg.webp)
"Calculations were made using the wavelength-dependent complex refractive indices of silicate (Draine 1985), glassy carbon (Edoh 1983), and Tholin (Khare et al. 1984). [...] these materials were chosen as broadly representative of the types of matter thought to be present in comet dust."[11]
"Observations using the Atacama Large Millimeter/submillimeter Array (ALMA) have revealed an unexpected spiral structure in the material around the old star R Sculptoris. This feature has never been seen before and is probably caused by a hidden companion star orbiting the star. This slice through the new ALMA data reveals the shell around the star, which shows up as the outer circular ring, as well as a very clear spiral structure in the inner material."[24] The image band is centered at 870 µm.
Meteorites
"The Raman spectra of some [interplanetary dust particle] IDPs also show red photoluminescence that is similar to the excess red emission seen in some astronomical objects and that has also been attributed to [polycyclic aromatic hydrocarbons] PAH s and hydrogenated amorphous carbon. Moreover, a part of the carbonaceous phase in IDPs and meteorites contains deuterium to hydrogen ratios that are greater than those for terrestrial samples."[25]
Plasma objects
The Hessdalen Light HL is an unexplained light usually seen in the Hessdalen valley in the municipality of Holtålen in Sør-Trøndelag county, Norway.
"HL [may be] formed by a cluster of macroscopic Coulomb crystals in a plasma produced by the ionization of air and dust by alpha particles during radon decay in the dusty atmosphere."[26]
"HL are characterized mostly by white color and sometimes by red color. It occurs mostly at night, more often in the winter season and with a peak around midnight."[26]
Gaseous objects
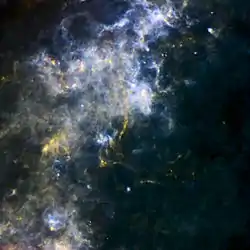
"This image [at right] from the Herschel Observatory reveals some of the coldest and darkest material in our galaxy. The choppy clouds of gas and dust pictured here are just starting to condense into new stars. The yellow filaments show the coldest dust dotted with the youngest embryonic stars."[27]
"Infrared, or submillimeter, light with a wavelength of 250 microns is represented in blue; 350-micron light in green; and 500-micron light in red. Much of this region of our galaxy would be hidden in visible-light views."[27]
"The area pictured is in the plane of our Milky Way galaxy, 60 degrees from the center. It spans a region 2.1 by 2.1 degrees. This image was taken by Herschel's spectral and photometric imaging receiver."[27]
Liquid objects
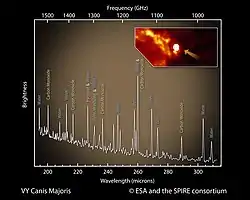
"This is one of the early spectra obtained with the SPIRE fourier transform spectrometer on Herschel. Shown here is a portion of the SPIRE spectrum of VY Canis Majoris (VY CMa), a red supergiant star near the end of its life, which is ejecting huge quantities of gas and dust into interstellar space. The inset is a SPIRE camera map of VY CMa, in which it appears as a bright compact source near the edge of a large extended cloud."[28]
"The VY CMa spectrum is amazingly rich, with prominent features from carbon monoxide (CO) and water (H2O). More than 200 other spectral features have been identified so far in the full spectrum, and several unidentified features are being investigated. Many of the features are due to water, showing that the star is surrounded by large quantities of hot steam. Observations like these will help to establish a detailed picture of the mass loss from stars and the complex chemistry occurring in their extended envelopes. As in all of the SPIRE spectra, the underlying emission increases towards shorter wavelengths, and is due to the emission from dust grains. The shape of the dust spectrum provides information on the properties of the dust."[28]
"VY Canis Majoris (VY CMa) is a red supergiant star located about 4900 light years from Earth in the constellation Canis Major. It is the largest known star, with a size of 2600 solar radii, and also one of the most luminous, with a luminosity in excess of 100 000 times that of the Sun. The mass of VY CMa lies in the range 30-40 solar masses, and it has a mass-loss rate of 2 x 10-4 solar masses per year."[28]
"The shell of gas it has ejected displays a complex structure; the circumstellar envelope is among the most remarkable chemical laboratories known in the Universe, creating a rich set of organic and inorganic molecules and dust species. Through stellar winds, these inorganic and organic compounds are injected into the interstellar medium, from which new stars orbited by new planets may form. Most of the carbon supporting life on Earth was forged by this kind of evolved star. VY CMa truly is a spectacular object, it is close to the end of its life and could explode as a supernova at any time."[28]
Rocky objects
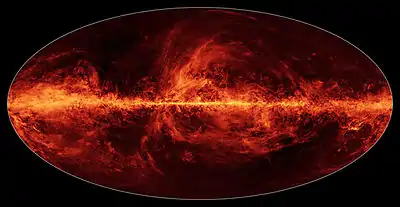
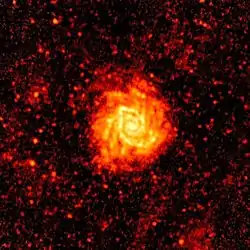
The Askaryan effect is the phenomenon whereby a particle traveling faster than the phase velocity of light in a dense dielectric (such as salt, ice or the lunar regolith) produces a shower of secondary charged particles which contain a charge anisotropy and thus emits a cone of coherent radiation in the radio or microwave part of the electromagnetic spectrum. It is similar to the Cherenkov effect.
"The red [image at center shows] the heat coming from dust throughout the Milky Way galaxy. Planck can capture this thermal light even though the dust is extremely cold — about minus 420 Fahrenheit (minus 251 Celsius)."[29]
"On 24 June 2009, SPIRE recorded its first images during the in-orbit commissioning phase of the Herschel mission. This picture, made before fine-tuning or in-orbit final calibration was performed, shows a SPIRE image of the galaxy M74 at a wavelength of 250 microns. The image traces emission by dust in clouds where star formation are active, and the nucleus and spiral arms show up clearly. Dust is part of the interstellar material fuelling star formation, and this image effectively shows the reservoirs of gas and dust that are available to be turned into stars in the galaxy. Significantly, the image frame is also filled with many other galaxies which are much more distant and only show up as point sources. There are also some extended structures, possibly due to clouds of dust in our own galaxy."[30]
"M74 (also known as NGC 628) is a face-on spiral galaxy located about 24 million light-years from Earth in the constellation Pisces. Visible light, produced mainly by the stars within the galaxy, reveals a bright nucleus and well-defined spiral arms that contain many small, bright regions where young massive stars have formed recently. The submillimetre SPIRE image traces the cold dust between the stars, and the spiral arms appear much more enhanced. This galaxy also contains many faint dots that are actually distant galaxies in the background and dust radiating at submillimetre wavelengths but are too distant for the structure in the galaxies to be resolved."[30]
Sun
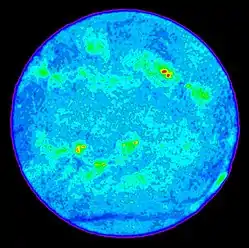
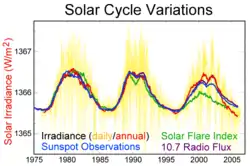
The parts of the Sun above the photosphere are referred to collectively as the solar atmosphere.[31] They can be viewed with telescopes operating across the electromagnetic spectrum, from radio through visible light to gamma rays, and comprise five principal zones: the temperature minimum, the chromosphere, the transition region, the corona, and the heliosphere.[31]
At right is a radio image of the Sun at 4.6 GHz. "The brightest discrete radio source is the Sun, but it is much less dominant than it is in visible light. The radio sky is always dark, even when the Sun is up, because atmospheric dust doesn't scatter radio waves, whose wavelengths are much longer than the dust particles."[32]
"The quiet Sun at 4.6 GHz imaged by the [Very Large Array] VLA with a resolution of 12 arcsec, or about 8400 km on the surface of the Sun. The brightest features (red) in this false-color image have brightness temperatures ~ 106 K and coincide with sunspots. The green features are cooler and show where the Sun's atmosphere is very dense. At this frequency the radio-emitting surface of the Sun has an average temperature of 3 x 104 K, and the dark blue features are cooler yet. The blue slash crossing the bottom of the disk is a feature called a filament channel, where the Sun's atmosphere is very thin: it marks the boundary of the South Pole of the Sun. The radio Sun is somewhat bigger than the optical Sun: the solar limb (the edge of the disk) in this image is about 20000 km above the optical limb."[32]
Direct measurements of radio emissions from the Sun at 10.7 cm also provide a proxy of solar activity [at second right] that can be measured from the ground since the Earth's atmosphere is transparent at this wavelength.
The general direction of the solar apex is southwest of the star Vega near the constellation of Hercules. There are several coordinates for the solar apex. The visual coordinates (as obtained by visual observation of the apparent motion) [are] right ascension (RA) 18h 28m 0s and declination (dec) of 30° North (in galactic coordinates: 56.24° longitude, 22.54° latitude). The radioastronomical position is RA 18h 03m 50.2s and dec 30° 00′ 16.8″ (galactic coordinates: 58.87° longitude, 17.72° latitude).
Dust storms
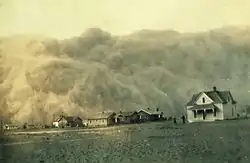
Weather is the state of the atmosphere, to the degree that it is hot or cold, wet or dry, calm or stormy, clear or cloudy.[33] Most weather phenomena occur in the troposphere,[34][35] just below the stratosphere. Weather refers, generally, to day-to-day temperature and precipitation activity, whereas climate is the term for the average atmospheric conditions over longer periods of time.[36]
On Earth, common weather phenomena include wind, cloud, rain, snow, fog and dust storms such as the one imaged on the right. Less common events include natural disasters such as tornadoes, hurricanes, typhoons and ice storms.
Zodiacal Lights
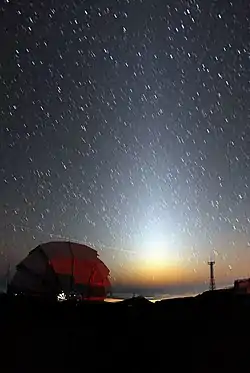
Chemical ions above the Earth's atmosphere, moving at very high speeds and at concentrations up to 100 particles per cm3 (centimeter cubed, a unit of volume) constitute the interplanetary medium.
The Zodiacal light is a faint, roughly triangular, diffuse white glow seen in the night sky that appears to extend up from the vicinity of the Sun along the ecliptic or zodiac.[37] It is best seen just after sunset and before sunrise in spring and autumn when the zodiac is at a steep angle to the horizon. Caused by sunlight scattered by space dust in the zodiacal cloud, it is so faint that either moonlight or light pollution renders it invisible. The zodiacal light decreases in intensity with distance from the Sun, but on very dark nights it has been observed in a band completely around the ecliptic. In fact, the zodiacal light covers the entire sky, being responsible for major part[38] of the total skylight on a moonless night. There is also a very faint, but still slightly increased, oval glow directly opposite the Sun which is known as the gegenschein. The dust forms a thick pancake-shaped cloud in the Solar System collectively known as the zodiacal cloud, which occupies the same plane as the ecliptic. The dust particles are between 10 and 300 micrometres in diameter, with most mass around 150 micrometres.[39]
Moon
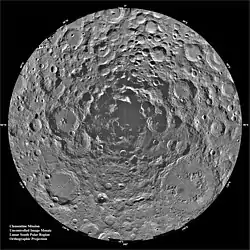
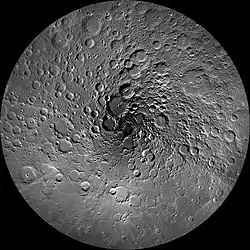
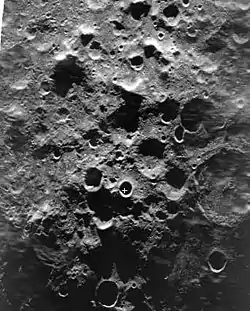

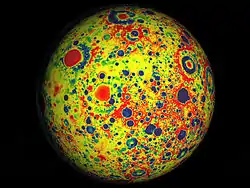
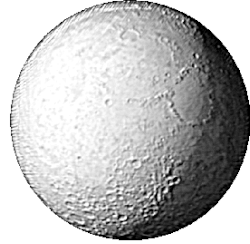
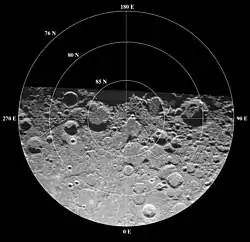
"The moon is comparatively close and was detected by radar, soon after the invention of the technique, in 1946.[40][41] Measurements included surface roughness and later mapping of shadowed regions near the poles.
"Very precise microwave measurements between two spacecraft, named Ebb and Flow, were used to map gravity with high precision and high spatial resolution. The field shown resolves blocks on the surface of about 12 miles (20 kilometres) and measurements are three to five orders of magnitude improved over previous data. Red corresponds to mass excesses and blue corresponds to mass deficiencies. The map shows more small-scale detail on the far side of the moon compared to the nearside because the far side has many more small craters."[42]
"Twin NASA probes orbiting Earth's moon have generated the highest resolution gravity field map of any celestial body. The new map, created by the Gravity Recovery and Interior Laboratory (GRAIL) mission, is allowing scientists to learn about the moon's internal structure and composition in unprecedented detail. Data from the two washing machine-sized spacecraft also will provide a better understanding of how Earth and other rocky planets in the solar system formed and evolved."[42]
"The gravity field map reveals an abundance of features never before seen in detail, such as tectonic structures, volcanic landforms, basin rings, crater central peaks and numerous simple, bowl-shaped craters. Data also show the moon's gravity field is unlike that of any terrestrial planet in our solar system."[43]
""What this map tells us is that more than any other celestial body we know of, the moon wears its gravity field on its sleeve," said GRAIL Principal Investigator Maria Zuber of the Massachusetts Institute of Technology in Cambridge. "When we see a notable change in the gravity field, we can sync up this change with surface topography features such as craters, rilles or mountains.""[43]
"According to Zuber, the moon's gravity field preserves the record of impact bombardment that characterized all terrestrial planetary bodies and reveals evidence for fracturing of the interior extending to the deep crust and possibly the mantle. This impact record is preserved, and now precisely measured, on the moon. The probes revealed the bulk density of the moon's highland crust is substantially lower than generally assumed. This low-bulk crustal density agrees well with data obtained during the final Apollo lunar missions in the early 1970s, indicating that local samples returned by astronauts are indicative of global processes."[43]
""With our new crustal bulk density determination, we find that the average thickness of the moon's crust is between 21 and 27 miles (34 and 43 kilometres), which is about 6 to 12 miles (10 to 20 kilometres) thinner than previously thought," said Mark Wieczorek, GRAIL co-investigator at the Institut de Physique du Globe de Paris. "With this crustal thickness, the bulk composition of the moon is similar to that of Earth. This supports models where the moon is derived from Earth materials that were ejected during a giant impact event early in solar system history.""[43]
"The map was created by the spacecraft transmitting radio signals to define precisely the distance between them as they orbit the moon in formation. As they fly over areas of greater and lesser gravity caused by visible features, such as mountains and craters, and masses hidden beneath the lunar surface, the distance between the two spacecraft will change slightly."[43]
""We used gradients of the gravity field in order to highlight smaller and narrower structures than could be seen in previous datasets," said Jeff Andrews-Hanna, a GRAIL guest scientist with the Colorado School of Mines in Golden. "This data revealed a population of long, linear gravity anomalies, with lengths of hundreds of kilometres, crisscrossing the surface. These linear gravity anomalies indicate the presence of dikes, or long, thin, vertical bodies of solidified magma in the subsurface. The dikes are among the oldest features on the moon, and understanding them will tell us about its early history.""[43]
"Clementine orbited the Moon in 1994 for 71 days, mapping the Moon globally in 11 wavelengths and measuring its topography by laser ranging. [... The] bistatic radar experiment (so-called because the spacecraft transmitted while we listened to the echoes on Earth) found evidence in the dark areas near the south pole of the Moon for material with high circular polarization ratio [CPR]".[44]
"Meanwhile, astronomers on Earth began publishing results questioning the Clementine and Lunar Prospector [1998-2000] results. With the giant Arecibo radiotelescope, radar images were taken from the Earth. They found radar reflections with high CPR lying in both permanent darkness and in sunlit areas. Ice is not stable in sunlight, so they postulated that all high CPR is caused by surface roughness; if any ice is at the lunar poles, it must be in a finely disseminated form, invisible to radar mapping."[44]
The experiment from Clemintine "was bistatic, i.e., the transmitter and receiver were in different places. Bistatic radar has the advantage of observing reflections through the phase angle, the angle between transmitted and received radio rays [...]. This phase dependence is important. It’s similar to the effect one gets from looking at a bicycle reflector at just the right angle: at certain angles, the internal planes in the transparent plastic align and a very bright reflection is seen. Similarly, in both radio and visible wavelengths on the Moon, we see an “opposition surge”, an apparent increase in brightness looking directly down from the sun (zero phase). Clementine orbited the Moon such that we could observe its phase dependence [...] and we specifically looked for this “opposition surge”, called the Coherent Backscatter Opposition Effect (CBOE). CBOE is particularly valuable to identify ice on planetary surfaces."[44]
"Clementine transmitted right circular polarized (RCP) radio and we listened on Earth in both right- and left-circular polarized (LCP) channels. The ratio of power received in these two channels is called the circular polarization ratio (CPR). The dry, equatorial Moon has CPR less than one, but the icy satellites of Jupiter all have CPR greater than one. We know these objects have surfaces of water ice; in this case, the ice acts as a radio-transparent media in which waves penetrate the ice, are scattered and reflected multiple times, and returned such that some of the waves are received in the same polarization sense as they are sent—they have CPR greater than unity"[44]
"The problem with CPR alone is that we can also get high values from very rough surfaces, such as a rough, blocky lava flow, which has angles that form many small corner reflectors. In this case, a radio wave could hit a rock face (changing RCP into LCP) and then bounce over to another rock face (changing the LCP back into RCP) and hence to the receiver [...]. This “double-bounce” effect also creates high CPR in that “same sense” reflections could mimic the enhanced CPR one gets from ice targets."[44]
At lower right is an image using the Goldstone DSS-14 antenna as a transmitter and the DSS-13 as a receiver, a form of radar interferometry. The cross for the south pole in the Arecibo image is in the Shackleton crater of the Goldstone image.
At fourth right is an image of the Moon using its thermal emission at 850 microns.
"The Moon and planets are not detectable by reflected solar radiation at radio wavelengths. However, they all emit thermal radiation, and Jupiter is a strong nonthermal source as well. If the Sun were suddenly switched off, the planets would remain radio sources for a long time, slowly fading as they cooled. At first glance, the λ = 0.85 mm radio image of the Moon [at second right] looks familiar, but there are differences from the visible Moon."[32]
"The darker right edge of the Moon is not being illuminated by the Sun, but it still emits radio waves because it does not cool to absolute zero during the lunar night. A subtler point is that the radio emission is not produced at the visible surface; it emerges from a layer about ten wavelengths thick. As a result, monthly temperature variations of the Moon decrease with increasing wavelength. These wavelength-dependent temperature variations encode information about the conductivity and heat capacity of the rocky and dusty outer layers of the Moon."[32]
"Radar images like the one [at fifth right] were recently used to search for water ice trapped in cold craters near the lunar poles."[32]
The ESA Lunar Lander Mission Lunar Dust Environment and Plasma Package: "Observe radio spectrum (with an additional goal to prepare for future radiation astronomy activities.)"[45]
Mars
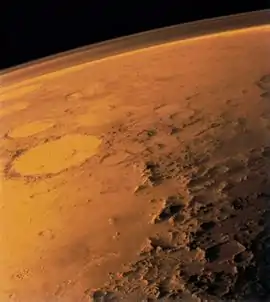
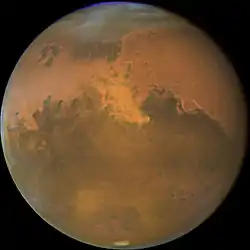
Mars is the fourth planet from the Sun in the Solar System. Named after the Roman god of war, Mars, it is often described as the "Red Planet" as the iron oxide prevalent on its surface gives it a reddish appearance.[46] The red-orange appearance of the Martian surface is caused by iron(III) oxide, more commonly known as hematite, or rust.[47] Much of the surface is deeply covered by finely grained iron(III) oxide dust.[48][49]
Comets
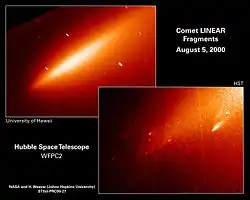
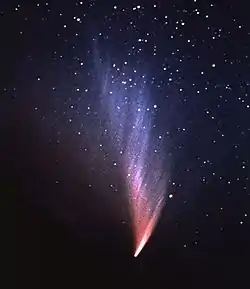
"My first thought was Hubble Space Telescope does it again! We caught the fish! This is amazing, very exciting, very neat."[50]
"Actually, I would have been more amazed if Hubble saw no pieces ... They just had to be there. The amount of heat available from sunlight just isn't enough to boil away something the size of a mountain in so short a time".[51]
"On July 27th, ground-based observers had lost sight of the bright core of the comet and were suggesting that the nucleus had totally disintegrated into a pile of dust. ... On Weaver's screen was at least a half dozen "mini-comets" with tails, resembling the shower of glowing fireballs from an aerial firework. They are clustered in the lance-head tip of an elongated stream of dust seen from a ground-based telescope."[52]
At left is an image of Comet West. "Comet West was a stunning sight in the predawn sky of March, 1976, bright with a tall and broad dust tail. ... [T]he comet [was] discovered on photographs taken in August 1975 by Richard West of the European Southern Observatory ... Comet West passed perihelion on February 25, 1976, at a distance of 0.20 a.u. [and] had reached about magnitude -3 at perihelion. Several observers saw it telescopically in daylight, and John Bortle observed it with the naked eye shortly before sunset. ... The following morning, March 7, ... It was brilliant, with a head as bright as Vega (which was nearly overhead) and a huge tail, about 20 degrees tall, straight near the bottom and bending to the left in its upper reaches. The comet quickly faded during March".[53]
"The λλ6300, 6363 Auroral red doublet of [OI] has been measured on digital sky-subtracted spectra of nine cometary nuclei ... The cometary oxygen lines are confined to their nuclear source, so that small apertures include much of the oxygen emission, particularly for small comets with Δ ≳ 1.0 AU."[54]
Interstellar medium
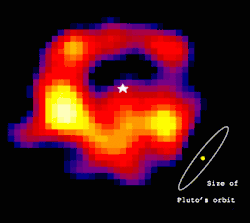
The submillimeter "wavelength view [at right] of a ring of dust particles around Epsilon Eridani, taken with the SCUBA camera at the James Clerk Maxwell Telescope. The false-colour scale is brightest where there is more dust. Epsilon Eridani is marked by the star symbol, although the star itself is not seen at submillimetre wavelengths. Pluto's orbit (marking the edge of our Solar System) is shown at the same scale."[55]
"The ring is "strikingly similar" to the outer comet zone in our Solar System, and shows an intriguing bright region that may be particles trapped around a young planet."[55]
"What we see looks just like the comet belt on the outskirts of our Solar System, only younger, [...] It's the first time we've seen anything like this around a star similar to our Sun. In addition, we were amazed to see a bright spot in the ring, which may be dust trapped in orbit around a planet."[55]
"Epsilon Eridani is far more similar to our Sun than either Vega or Fomalhaut."[55]
"This star system is a strong candidate for planets, but if there are planets, it's unlikely there could be life yet. When the Earth was this young, it was still being very heavily bombarded by comets and other debris."[55]
"It is also a star in our local neighbourhood, being only about 10 light years away, which is why we can see so much detail in the new image."[55]
"If an astronomer could have seen what our Solar System looked like four billion years ago, it would have been very much as Epsilon Eridani looks today, [...] This is a star system very like our own, and the first time anyone has found something that truly resembles our Solar System; it's one thing to suspect that it exists, but another to actually see it, and this is the first observational evidence."[56]
"Beyond Pluto in our Solar System is a region containing more than 70,000 large comets, and hundreds of millions of smaller ones, called the "Kuiper belt". The image [...] shows dust particles that the astronomers believe are analogous to our Kuiper belt at the same distance from Epsilon Eridani as the Kuiper belt is from our Sun. Although the image cannot reveal comets directly, the dust that is revealed is believed to be debris from comets."[55]
"Epsilon Eridani's inner region contains about 1,000 times more dust than our Solar System's inner region, which may mean it has about 1,000 times more comets [...]. Epsilon Eridani is believed to be only 500 million years to 1 billion years old; our Sun is estimated to be 4.5 billion years old, and its inner region is believed to have looked very similar at that age."[55]
"The new image -- which is from short-radio wavelengths, and is not an optical picture -- was obtained using the 15-meter James Clerk Maxwell Telescope [JCMT] at the Mauna Kea Observatory in Hilo, Hawaii. The JCMT is the world's largest telescope dedicated to the study of light at "submillimeter" wavelengths. The [...] camera called SCUBA (Submillimeter Common User Bolometer Array), which was built by the Royal Observatory in Edinburgh (which is now the UK Astronomical Technology Centre). SCUBA uses detectors cooled to a tenth of a degree above absolute zero (-273 degrees Celsius) to measure the tiny amounts of heat emission from small dust particles at a wavelength close to one-millimeter."[55]
"The implication is that if there is one system similar to ours at such a close star, presumably there are many others, [...] In the search for life elsewhere in the universe, we have never known where to look before. Now, we are closing in on the right candidates in the search for life."[56]
"A region near the star that is partially evacuated indicates that planets may have formed, [...] the presence of planets is the most likely explanation for the absence of dust in this region because planets absorb the dust when they form."[55]
"There may be a planet stirring up the dust in the ring and causing the bright spot, or it could be the remnants of a massive collision between comets."[57]
Molecular clouds
G0.253+0.016 was probed "with another network of telescopes, the Combined Array for Research in Millimeter-wave Astronomy [CARMA] in California."[58]
"G0.253+0.016, which is about 30 light-years long, defies the conventional wisdom that dense gas glouds should produce lots of stars. ... The cloud is 25 times more dense than the famous Orion Nebula, which is birthing stars at a furious rate. But only a few stars are being born in G0.253+0.016, and they're pretty much all runts."[58]
"It's a very dense cloud and it doesn't form any massive stars, which is very weird"[58].
"CARMA data showed that gas within G0.253+0.016 is zipping around 10 times faster than gas in similar clouds. G0.253+0.016 is on the verge of flying apart, with its gas churning too violently to coalesce into stars. Further, the ... cloud is full of silicon monoxide, a compound typically produced when fast-moving gas smashes into dust particles. The abnormally large amounts of silicon monoxide suggest that G0.253+0.016 may actually consist of two colliding clouds, whose impact is generating powerful shockwaves."[58]
When surveyed at 1.1 mm as part of the Bolocam Galactic Plane Survey, "[t]he only currently known starless [massive proto-cluster] MPC is G0.253+0.016, which lies within the dense central molecular zone and is subject to greater environmental stresses than similar objects in the Galactic plane (Longmore et al. 2012)."[59]
Star-forming regions
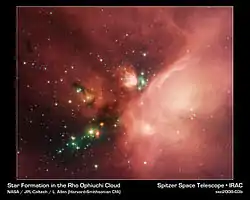
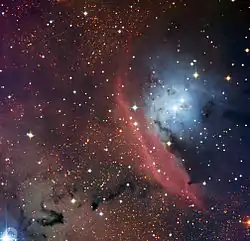
"Newborn stars peek out from beneath their natal blanket of dust in this dynamic image of the Rho Ophiuchi dark cloud [in the image at right] from NASA's Spitzer Space Telescope. Called "Rho Oph" by astronomers, it's one of the closest star-forming regions to our own solar system. Located near the constellations Scorpius and Ophiuchus, the nebula is about 407 light years away from Earth."[60]
"Rho Oph is a complex made up of a large main cloud of molecular hydrogen, a key molecule allowing new stars to form from cold cosmic gas, with two long streamers trailing off in different directions. Recent studies using the latest X-ray and infrared observations reveal more than 300 young stellar objects within the large central cloud. Their median age is only 300,000 years, very young compared to some of the universe's oldest stars, which are more than 12 billion years old."[60]
"This false-color image of Rho Oph's main cloud, Lynds 1688, was created with data from Spitzer's infrared array camera, which has the highest spatial resolution of Spitzer's three imaging instruments. Blue represents 3.6 micron light, green is 4.5 micron light, orange is 5.8, and red is 8.0. The multiple wavelengths reveal different aspects of the dust surrounding and between the embedded stars, yielding information about the stars and their birthplace."[60]
"The colors in this image reflect the relative temperatures and evolutionary states of the various stars. The youngest stars are surrounded by dusty disks of gas from which they, and their potential planetary systems, are forming. These young disk systems show up as yellow-green tinted stars in this image. Some of these young stellar objects are surrounded by their own compact nebulae. More evolved stars, which have shed their natal material, are blue-white."[60]
"The gas in the clouds of NGC 6559, mainly hydrogen, is the raw material for star formation. When a region inside this nebula gathers enough matter, it starts to collapse under its own gravity. The center of the cloud grows ever denser and hotter, until thermonuclear fusion begins and a star is born. The hydrogen atoms combine to form helium atoms, releasing energy that makes the star shine. In regions where it is very dense, the dust completely blocks the light behind it, as is the case for the dark isolated patches and sinuous lanes to the bottom left-hand side and right-hand side of the image".[61]
"The Danish 1.54-metre telescope located at ESO’s La Silla Observatory in Chile has captured a striking image of NGC 6559, an object that showcases the anarchy that reigns when stars form inside an interstellar cloud. This region of sky includes glowing red clouds of mostly hydrogen gas, blue regions where starlight is being reflected from tiny particles of dust and also dark regions where the dust is thick and opaque."[62]
"The two colors of the cloud represent a pair of nebulas. Once the young stars are born, they "energize" the hydrogen surrounding them, ESO officials said. The gas then creates the red wispy cloud — known to astronomers as an "emission nebula" — in the center of the image."[63]
"These young stars are usually of spectral type O and B, with temperatures between 10 000 and 60 000 K, which radiate huge amounts of high energy ultraviolet light that ionises the hydrogen atoms."[64]
"The blue section of the photo — representing a "reflection nebula" — shows light from the newly formed stars in the cosmic nursery being reflected in all directions by the particles of dust made of iron, carbon, silicon and other elements in the interstellar cloud."[63]
NGC 6559 is planetary nebula located at a distance of about 5000 light-years from Earth, in the constellation of Sagittarius.
"NGC 6559 is a cloud of gas and dust located at a distance of about 5000 light-years from Earth, in the constellation of Sagittarius (The Archer). The glowing region is a relatively small object, just a few light-years across, in contrast to the one hundred light-years and more spanned by its famous neighbour, the Lagoon Nebula (Messier 8, eso0936). Although it is usually overlooked in favour of its distinguished companion, NGC 6559 has the leading role in this new picture."[64]
"The Milky Way fills the background of the image with countless yellowish older stars. Some of them appear fainter and redder because of the dust in NGC 6559."[64]
"This eye-catching image of star formation was captured by the Danish Faint Object Spectrograph and Camera (DFOSC)".[64]
Brown Dwarfs
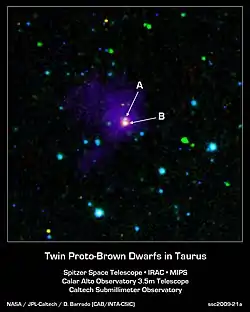
"This image [at right] shows two young brown dwarfs, objects that fall somewhere between planets and stars in terms of their temperature and mass. Brown dwarfs are cooler and less massive than stars, never igniting the nuclear fires that power their larger cousins, yet they are more massive (and normally warmer) than planets. When brown dwarfs are born, they heat the nearby gas and dust, which enables powerful infrared telescopes like NASA's Spitzer Space Telescope to detect their presence."[65]
"Here we see a long sought-after view of these very young objects, labeled as A and B, which appear as closely-spaced purple-blue and orange-white dots at the very center of this image. The surrounding envelope of cool dust surrounding this nursery can be seen in purple."[65]
"These twins, which were found in the region of the Taurus-Auriga star-formation complex, are the youngest of their kind ever detected. They are also helping astronomers solve a long-standing riddle about how brown dwarfs are formed more like stars or more like planets? Based on these findings, the researchers think they have found the answer: Brown dwarfs form like stars."[65]
"This image combined data from three different telescopes on the ground and in space. Near-infrared observations collected at the Calar Alto Observatory in Spain cover wavelengths of 1.3 and 2.2 microns (rendered as blue). Spitzer's infrared array camera contributed the 4.5-micron (green) and 8.0-micron (yellow) observations, and its multiband imaging photometer added the 24-micron (red) component. The Caltech Submillimeter Observatory in Hawaii made the far-infrared observations at 350 microns (purple)."[65]
CW Leonis
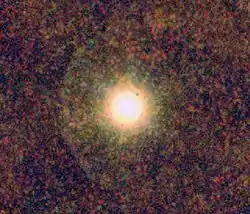
"This Herschel image shows IRC+10216, also known as CW Leonis -- a star rich in carbon where astronomers were surprised to find water. This color-coded image shows the star, surrounded by a clumpy envelope of dust, at three infrared wavelengths, taken by Herschel's spectral and photometric imaging receiver (SPIRE) and photodetector array camera and spectrometer (PACS). Blue shows light of 160 microns; green shows 250 microns; and red shows 350 microns."[66]
IK Tauri
"For both distributions, there is a dust shell of radius about 100 mas, or diameter of 200 mas, and two more or less discrete shells separated by about 240 mas. This approximately regular spacing is what produces a hump in the visibility curve in the (6-8.5) x 105 rad-1 range, though in somewhat different positions for the two years. It can be seen from Figure 4 that the prominent and well-defined outer dust shell expanded between 1992 and 1993. One should not expect that each dust shell emitted has exactly the same velocity, but if the motion of the outer shell is taken as 20.5 km s-1, an average of the 22 km s-1 velocity measured by CO line emission of material surrounding the star (Knapp & Morris 1985) and of 18.7 km s-1 for OH masers (Bowers et al. 1989), then the stellar distance can be obtained from the observed motion. The displacement of the outer shell is 17 mas between average dates of 1992 August 24 and 1993 September 14. From these values, the stellar distance is calculated to be 265 pc. This is in good agreement with the estimates of 270 and 220 pc by Knapp & Morris (1985) and Le Sidaner & Le Bertre (1996), respectively. Other estimates in the literature for the distance to IK Tau vary from 240 to 500 pc (Knapp & Morris 1985). If the velocity of the outer shell is indeed close to the average of OH and CO gas measured by microwave astronomy, the distance of 265 pc should be approximately correct and should confirm the distance estimates that have arrived at comparable values, rather than others that are substantially different."[67]
Trifid Nebula
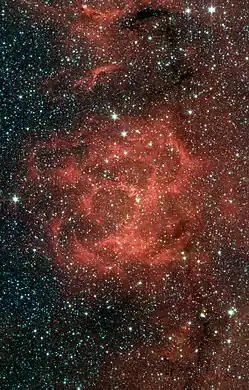
"The glowing Trifid Nebula [in the image at right] is revealed in an infrared view from NASA's Spitzer Space Telescope. The Trifid Nebula is a giant star-forming cloud of gas and dust located 5,400 light-years away in the constellation Sagittarius."[68]
"The false-color Spitzer image reveals a different side of the Trifid Nebula. Where dark lanes of dust are visible trisecting the nebula in a visible-light picture, bright regions of star-forming activity are seen in the Spitzer picture. All together, Spitzer uncovered 30 massive embryonic stars and 120 smaller newborn stars throughout the Trifid Nebula, in both its dark lanes and luminous clouds. These stars are visible in the Spitzer image, mainly as yellow or red spots. Embryonic stars are developing stars about to burst into existence."[68]
"Ten of the 30 massive embryos discovered by Spitzer were found in four dark cores, or stellar "incubators," where stars are born. Astronomers using data from the Institute of Radioastronomy millimeter telescope in Spain had previously identified these cores but thought they were not quite ripe for stars. Spitzer's highly sensitive infrared eyes were able to penetrate all four cores to reveal rapidly growing embryos."[68]
"Astronomers can actually count the individual embryos tucked inside the cores by looking closely at this Spitzer image taken by its infrared array camera (IRAC). This instrument has the highest spatial resolution of Spitzer's imaging cameras. The embryos are thought to have been triggered by a massive "type O" star, which can be seen as a white spot at the center of the nebula. Type O stars are the most massive stars, ending their brief lives in explosive supernovas. The small newborn stars probably arose at the same time as the O star, and from the same original cloud of gas and dust."[68]
"This Spitzer mosaic image uses data from IRAC showing light of 3.6 microns (blue), 4.5 microns (green), 5.8 microns (orange) and 8.0 microns (red)."[68]
Orion
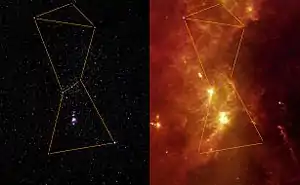
{{free media}}
"Seen here [on the right] is a comparison of the constellation Orion viewed in visible and infrared light. In the infrared image from NASA’s IRAS mission we can see clouds of dust and gas invisible to the human eye. The bright spots are the locations where stars are being born."[69]
Milky Way
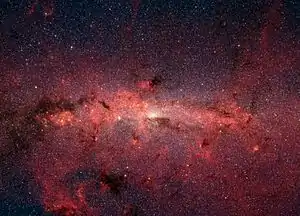
{{free media}}
Of the total mass of the galaxy's stars, interstellar dust accounts for an additional 1% of the total mass of the gas.[70]
Dust rings
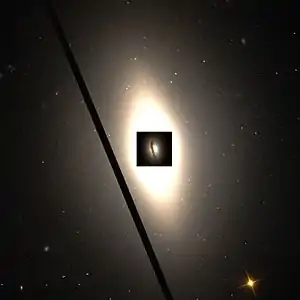
{{free media}}
NGC 4435 is a barred lenticular galaxy currently interacting with NGC 4438 with a detected dust ring around its nucleus as shown in the imade on the right. Studies of the galaxy by the Spitzer Space Telescope revealed a relatively young (190 million years) stellar population within the galaxy's nucleus, which may have originated through the interaction with NGC 4438 compressing gas and dust in that region, triggering a starburst.[71] It also has a long tidal tail possibly caused by the interaction with the mentioned galaxy;[72] however, other studies suggest that tail is actually a galactic cirrus in the Milky Way totally unrelated to NGC 4435.[73]
Whirlpool Galaxy
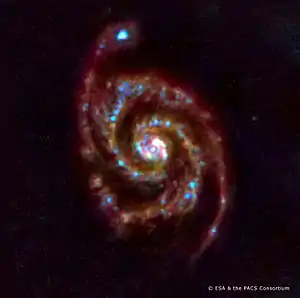
{{free media}}
Far-infrared astronomy deals with objects visible in far-infrared radiation (extending from 30 µm towards submillimeter wavelengths around 450 µm).
"Red, green and blue correspond to the 160-micron, 100-micron and 70-micron wavelength bands of the Herschels Photoconductor Array Camera and Spectrometer, PACS instruments [in the far-infrared image on the right]."[74]
"Glowing light from clouds of dust and gas around and between the stars is visible clearly. These clouds are a reservoir of raw material for ongoing star formation in this galaxy. Blue indicates regions of warm dust that is heated by young stars, while the colder dust shows up in red."[74]
Starburst galaxy
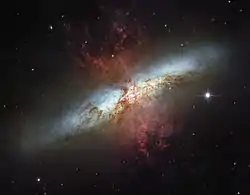
"The presence of ERE has been established spectroscopically in ... the starburst galaxy M82 (Perrin, Darbon, & Sivan 1995)."[75]
"This mosaic image [at right] is the sharpest wide-angle view ever obtained of M82. The galaxy is remarkable for its bright blue disk, webs of shredded clouds, and fiery-looking plumes of glowing hydrogen blasting out of its central regions."[76]
"Throughout the galaxy's center, young stars are being born 10 times faster than they are inside our entire Milky Way Galaxy. The resulting huge concentration of young stars carved into the gas and dust at the galaxy's center. The fierce galactic superwind generated from these stars compresses enough gas to make millions of more stars."[76]
"In M82, young stars are crammed into tiny but massive star clusters. These, in turn, congregate by the dozens to make the bright patches, or "starburst clumps," in the central parts of M82. The clusters in the clumps can only be distinguished in the sharp Hubble images. Most of the pale, white objects sprinkled around the body of M82 that look like fuzzy stars are actually individual star clusters about 20 light-years across and contain up to a million stars."[76]
"The rapid rate of star formation in this galaxy eventually will be self-limiting. When star formation becomes too vigorous, it will consume or destroy the material needed to make more stars. The starburst then will subside, probably in a few tens of millions of years."[76]
"Located 12 million light-years away, M82 appears high in the northern spring sky in the direction of the constellation Ursa Major, the Great Bear. It is also called the "Cigar Galaxy" because of the elliptical shape produced by the oblique tilt of its starry disk relative to our line of sight."[76]
"The observation was made in March 2006, with the Advanced Camera for Surveys' Wide Field Channel. Astronomers assembled this six-image composite mosaic by combining exposures taken with four colored filters that capture starlight from visible and infrared wavelengths as well as the light from the glowing hydrogen filaments."[76]
Galaxies
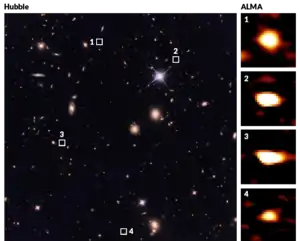
{{fairuse}}
Submillimetre "(wavelength 870 micrometres) detections of 39 massive star-forming galaxies at z > 3, which are unseen in the spectral region from the deepest ultraviolet to the near-infrared [have occurred]."[77]
The catalog entries that appear to correspond to numbers 1-4 in the image on the right are {COSMOS) COS-27285 (1) z = , COS-19762 (2) z = , COS-23718 (3) z = , and COS-25881 (4) z = .
Balloons
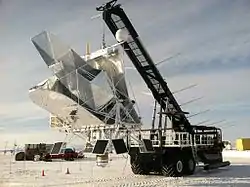
The Balloon-borne Large Aperture Submillimeter Telescope (BLAST) is a submillimeter telescope that hangs from a high altitude balloon. It has a 2 meter primary mirror that directs light into bolometer arrays operating at 250, 350, and 500 µm. ... BLAST's primary science goals are:[78]
- Measure photometric redshifts, rest-frame FIR luminosities and star formation rates of high-redshift starburst galaxies, thereby constraining the evolutionary history of those galaxies that produce the FIR/submillimeter background.
- Measure cold pre-stellar sources associated with the earliest stages of star and planet formation.
- Make high-resolution maps of diffuse galactic emission over a wide range of galactic latitudes.
High-altitude balloons and aircraft can get above much of the atmosphere. The BLAST experiment and SOFIA are two examples, respectively, although SOFIA can also handle near infrared observations.
At left above "NASA's balloon-carried BLAST sub-millimeter telescope is hoisted into launch position on Dec. 25, 2012, at McMurdo Station in Antarctica on a mission to peer into the cosmos."[79] The giant helium-filled balloon is slowly drifting about 36 km above Antarctica. It was "[l]aunched on Tuesday (Dec. 25) from the National Science Foundation's Long Duration Balloon (LDB) facility ... This is the fifth and final mission for BLAST, short for the Balloon-borne Large-Aperture Submillimeter Telescope. ... "BLAST found lots of so-called dark cores in our own Milky Way — dense clouds of cold dust that are supposed to be stars-in-the-making. Based on the number of dark cores, you would expect our galaxy to spawn dozens of new stars each year on average. Yet, the galactic star formation rate is only some four solar masses per year." So why is the stellar birth rate in our Milky Way so low? Astronomers can think of two ways in which a dense cloud of dust is prevented from further contracting into a star: turbulence in the dust, or the collapse-impeding effects of magnetic fields. On its new mission, BLAST should find out which process is to blame. ... [The 1800-kilogram] stratospheric telescope will observe selected star-forming regions in the constellations Vela and Lupus."[80]
See also
- Portal:Radiation astronomy
- Radiation astronomy/Courses
References
- ↑ PJ Ozer (1995). Fantechi, R.. ed. Lithometeors in relation with desertification in the Sahelian area of Niger, In: Desertification in a European context: physical and socio-economic aspects. Luxembourg: Office for Official Publications of the European Community. pp. 567-74. ISBN 92-827-4163-X. http://www.cabdirect.org/abstracts/19971901329.html. Retrieved 2013-02-17.
- 1 2 Mark R. Mireles; Kirth L. Pederson; Charles H. Elford (February 21, 2007). Meteorologial Techniques. Offutt Air Force Base, Nebraska, USA: Air Force Weather Agency/DNT. Retrieved 2013-02-17.
- ↑ Jian-qiao Yu; Xia1 Wang; Li Wen; Jing-shun Wang (April 2008). "Studies on Correlation of Heavy Metal Pollution in Soil, Lithometeor". Journal of Agricultural Science and Technology (04). http://en.cnki.com.cn/Article_en/CJFDTOTAL-NKDB200804025.htm. Retrieved 2013-02-17.
- ↑ P. Ozer (1998). G. Demaree; J. Alexandre; M. de Dapper (eds.). Lithometeors and wind velocity in relation with desertification during the dry season from 1951 to 1994 in Niger, In: International Conference on tropical climatology, meteorology and hydrology in memoriam Franz Bultot. Bruxelles (Belgium): Royal Meteorological Institute of Belgium; Royal Academy of Overseas Sciences. pp. 212–27. Retrieved 2013-02-17.
- ↑ R.W. Alexander Jr; R.J. Bell; M.A. Ordal; L.L. Long; M. Querry (January 1, 1986). "Millimeter and submillimeter wavelength spectroscopy of natural minerals and metals". Applied Physics Communications 6 (1). http://www.osti.gov/energycitations/product.biblio.jsp?osti_id=6649643. Retrieved 2013-10-20.
- 1 2 3 4 5 6 Yacine Ali-Haïmoud (2013). "Spinning dust radiation: a review of the theory". Advances in Astronomy 2013 (462697). doi:10.1155/2013/462697. http://arxiv.org/pdf/1211.2748v1.pdf. Retrieved 2014-10-19.
- ↑ T.G. Phillips, J. Keene (November 1992). "Submillimeter astronomy [heterodyne spectroscopy"]. Proceedings of the IEEE 80 (11): 1662-78. doi:10.1109/5.175248. http://ieeexplore.ieee.org/xpls/abs_all.jsp?arnumber=175248. Retrieved 2013-10-21.
- 1 2 3 4 5 Jean-Charles Cuillandre (February 26, 2014). Image of the Day: The Snake Nebula --From Cosmic Seeds to Massive Stars. Daily Galaxy. Retrieved 2014-03-13.
- ↑ Ke Wang (February 26, 2014). Image of the Day: The Snake Nebula --From Cosmic Seeds to Massive Stars. Daily Galaxy. Retrieved 2014-03-13.
- 1 2 A. J. Barger; L. L. Cowie; D. B. Sanders (June 10, 1999). "Resolving the submillimeter background: the 850 micron galaxy counts". The Astrophysical Journal 518 (1): L5-8. doi:10.1086/312054. http://iopscience.iop.org/1538-4357/518/1/L5. Retrieved 2013-10-22.
- 1 2 David Jewitt; Jane Luu (November 1992). "Submillimeter Continuum Emission from Comets". Icarus 108 (1): 187-96. http://www.sciencedirect.com/science/article/pii/0019103592900286. Retrieved 2013-10-22.
- 1 2 eso1321a (May 15, 2013). An APEX view of star formation in the Orion Nebula. ESO. Retrieved 2014-03-14.
- ↑ Bill Keel (October 2003). Jets, Superluminal Motion, and Gamma-Ray Bursts. Tucson, Arizona USA: University of Arizona. Retrieved 2014-03-19.
- 1 2 Bill Keel (October 2003). The powerful radio galaxy Cygnus A. Tucson, Arizona USA: University of Arizona. Retrieved 2014-03-19.
- 1 2 3 4 5 6 7 I.A. Smith; R.P.J. Tilanus; J. van Paradijs; T.J. Galama; P.J. Groot; P. Vreeswijk; C. Kouveliotou; R.A.M. Wijers et al. (July 1999). "SCUBA sub-millimeter observations of gamma-ray bursters I. GRB 970508, 971214, 980326, 980329, 980519, 980703, 981220, 981226". Astronomy and Astrophysics 347 (07): 92-8. http://adsabs.harvard.edu/full/1999A%26A...347...92S. Retrieved 2013-10-22.
- ↑ R. Kraft (January 28, 2009). Centaurus A*. European Southern Observatory. Retrieved 2014-03-12.
- ↑ Bill Keel (October 2003). Galaxy, jet, and obscuring disk in NGC 1068. Tucson, Arizona USA: University of Arizona. Retrieved 2014-03-19.
- 1 2 3 Spitzersteph (November 4, 2010). Analyzing the Pieces of a Warped Galaxy. San Francisco, California: Wikimedia Foundation, Inc. Retrieved 2014-03-12.
- 1 2 3 4 Karl Stapelfeldt (December 18, 2003). Fomalhaut Circumstellar Disk. Pasadena, California USA: California Institute of Technology. Retrieved 2014-03-12.
- ↑ Hankwang (October 31, 2008). Terahertz radiation: Difference between revisions, In: Wikipedia. p. 1. Retrieved 2012-08-05.
- 1 2 3 4 Lucas Cieza; Luisa Rebull; Jes Jorgensen (October 25, 2006). Perseus' Stellar Neighbors. Pasadena, California USA: NASA/JPL/California Institute of Technology. Retrieved 2014-03-06.
- 1 2 T. L. Smith; A. N. Witt (December 1999). "The Photoluminescence Efficiency of Extended Red Emission as a Constraint for Interstellar Dust". Bulletin of the American Astronomical Society 31: 1479. http://adsabs.harvard.edu/abs/1999AAS...195.7406S. Retrieved 2013-08-02.
- ↑ Daniel T. Britt; Carle M. Pieters; Peter H. Schultz (December 1986). "Source of the optical red-slope in iron-rich meteorites". Meteoritics 21 (12): 340-1. http://adsabs.harvard.edu/full/1986Metic..21..340B. Retrieved 2013-08-02.
- ↑ M. Maercker; et al. (October 10, 2012). Curious spiral spotted by ALMA around red giant star R Sculptoris (data visualisation). Atacama, Chile: European Southern Observatory. Retrieved 2014-03-12.
- ↑ L. J. Allamandola; S. A. Sandford; B. Wopenka (July 3, 1987). "Interstellar Polycyclic Aromatic Hydrocarbons and Carbon in Interplanetary Dust Particles and Meteorites". Science 237 (4810): 56-9. doi:10.1126/science.237.4810.56. http://www.sciencemag.org/content/237/4810/56.short. Retrieved 2013-08-02.
- 1 2 G.S. Paiva, C.A. Taft (October 2010). "A hypothetical dusty plasma mechanism of Hessdalen lights". Journal of Atmospheric and Solar-Terrestrial Physics 72 (16): 1200-3. doi:10.1016/j.jastp.2010.07.022. http://www.sciencedirect.com/science/article/pii/S136468261000218X. Retrieved 2013-08-02.
- 1 2 3 nhsc2009 (October 2, 2009). Dark Wombs of Stars. Pasadena, California USA: California Institute of Technology. Retrieved 2014-03-12.
- 1 2 3 4 M. Groenewegen (November 27, 2009). SPIRE spectrum of VY Canis Majoris. Pasadena, California USA: Caltech. Retrieved 2014-03-12.
- ↑ Calla Cofield (6 February 2015). Spectacular Milky Way Maps Show Our Galaxy in New Light. Space.com. Retrieved 2015-11-16.
- 1 2 nhsc2009 (July 9, 2009). SPIRE image of M74 at 250 m. Pasadena, California USA: Caltech. Retrieved 2014-03-12.
- 1 2 Abhyankar, K.D. (1977). "A Survey of the Solar Atmospheric Models". Bull. Astr. Soc. India 5: 40–44. http://prints.iiap.res.in/handle/2248/510.
- 1 2 3 4 5 S.G. Djorgovski; et al. A Tour of the Radio Universe. National Radio Astronomy Observatory. Retrieved 2014-03-16.
- ↑ Merriam-Webster Dictionary. Weather. Retrieved on 27 June 2008.
- ↑ Glossary of Meteorology. Hydrosphere. Retrieved on 27 June 2008.
- ↑ Glossary of Meteorology. Troposphere. Retrieved on 27 June 2008.
- ↑ "Climate". Glossary of Meteorology. American Meteorological Society. Retrieved 14 May 2008.
- ↑ Internet Encyclopedia of Science Accessed April 2010
- ↑ Reach, W. T. (1997). "The structured zodiacal light: IRAS, COBE, and ISO observations", page 1 (in Introduction)
- ↑ Bernhard Peucker-Ehrenbrink; Birger Schmitz (2001). Accretion of Extraterrestrial Matter Throughout Earth's History. Springer. pp. 66–67. ISBN 0-306-46689-9.
- ↑ J. Mofensen (February, April 1946). "Radar Echoes from the Moon". Nature, Electronics 157, 19 (3379): 129, 92-8. doi:10.1038/157129b0.
- ↑ Z. Bay, Reflection of microwaves from the moon," Hung. Acta Phys., vol. 1, pp. 1-22; April, 1946.
- 1 2 Tony Greicius (December 6, 2012). GRAIL's Gravity Map of the Moon. NASA/JPL-Caltech/MIT/GSFC. Retrieved 2012-12-15.
- 1 2 3 4 5 6 PR Newswire (December 5, 2012). NASA Twin Spacecraft Create Most Accurate Gravity Map Of Moon. Retrieved 2012-12-15.
- 1 2 3 4 5 P. Spudis (November 6, 2006). Ice on the Moon. The Space Review. Retrieved 12 April 2007.
- ↑ B. Gardini (2011). "ESA strategy for human exploration and the Lunar Lander Mission". Memorie della Societa Astronomica Italiana 82: 422-29. http://adsabs.harvard.edu/full/2011MmSAI..82..422G. Retrieved 5 May 2019.
- ↑ The Lure of Hematite. NASA. March 28, 2001. Retrieved 2009-12-24.
- ↑ Mark Peplow. How Mars got its rust. MacMillan Publishers Ltd. Retrieved 2007-03-10.
- ↑ Philip R. Christensen, et al. (June 27, 2003). "Morphology and Composition of the Surface of Mars: Mars Odyssey THEMIS Results". Science 300 (5628): 2056–61. doi:10.1126/science.1080885. PMID 12791998.
- ↑ Matthew P. Golombek (June 27, 2003). "The Surface of Mars: Not Just Dust and Rocks". Science 300 (5628): 2043–2044. doi:10.1126/science.1082927. PMID 12829771.
- ↑ Harold Weaver (August 7, 2000). Hubble Discovers Missing Pieces of Comet Linear. Baltimore, Maryland USA: Hubblesite - Newscenter. Retrieved 2013-05-02.
- ↑ Carey Lisse (August 7, 2000). Hubble Discovers Missing Pieces of Comet Linear. Baltimore, Maryland USA: Hubblesite - Newscenter. Retrieved 2013-05-02.
- ↑ Ray Villard; Michael Purdy (August 7, 2000). Hubble Discovers Missing Pieces of Comet Linear. Baltimore, Maryland USA: Hubblesite - Newscenter. Retrieved 2013-05-02.
- ↑ Tony Hoffman. Comet West: The Great Comet of 1976. Earthlink. Retrieved 2013-05-02.
- ↑ Hyron Spinrad (December 1982). "Observations of the red auroral oxygen lines in nine comets". Publications of the Astronomical Society of the Pacific 94 (12): 1008-16. doi:10.1086/131101. http://www.jstor.org/stable/10.2307/40678099. Retrieved 2013-01-21.
- 1 2 3 4 5 6 7 8 9 10 Jane Greaves (July 8, 1998). Astronomers discover a nearby star system just like our own Solar System. Hilo, Hawaii, USA: Joint Astronomy Centre. Retrieved 2014-03-12.
- 1 2 Benjamin Zuckerman (July 8, 1998). Astronomers discover a nearby star system just like our own Solar System. Hilo, Hawaii, USA: Joint Astronomy Centre. Retrieved 2014-03-12.
- ↑ Bill Dent (July 8, 1998). Astronomers discover a nearby star system just like our own Solar System. Hilo, Hawaii, USA: Joint Astronomy Centre. Retrieved 2014-03-12.
- 1 2 3 4 SPACE.com Staff (January 14, 2013). Baffling Star Birth Mystery Finally Solved. Yahoo! Inc. Retrieved 2013-01-15.
- ↑ A. Ginsburg; E. Bressert; J. Bally; C. Battersby (October 20, 2012). "There are No Starless Massive Proto-Clusters in the First Quadrant of the Galaxy". The Astrophysical Journal Letters 758 (2): L29-33. doi:10.1088/2041-8205/758/2/L29. http://arxiv.org/pdf/1208.4097.pdf. Retrieved 2013-01-15.
- 1 2 3 4 G.G. Fazio; P.C. Myers; L. Allen; S.T. Megeath; E.T. Young; J. Muzerolle; N.J. Evans II; G.A. Blake; P.M. Harvey; D.W. Koerner; L.G. Mundy; D.L. Padgett; A.I. Sargent; K.R. Stapelfeldt; E.F. van Dishoeck (February 11, 2008). Young Stars in Their Baby Blanket of Dust. Pasadena, California USA: NASA/JPL/Caltech. Retrieved 2014-03-06.
- ↑ ESO officials (May 2, 2013). Dusty Star-Spawning Space Cloud Glows In Amazing Photo. La Silla, Chile: Yahoo! News. Retrieved 2013-05-02.
- ↑ eso1320a (May 2, 2013). The star formation region NGC 6559. La Silla Observatory, Chile: European Southern Observatory. Retrieved 2013-05-02.
- 1 2 Miriam Kramer (May 2, 2013). Dusty Star-Spawning Space Cloud Glows In Amazing Photo. La Silla, Chile: Yahoo! News. Retrieved 2013-05-02.
- 1 2 3 4 Richard Hook. An Anarchic Region of Star Formation. Garching bei München, Germany: European Southern Observatory. Retrieved 2013-05-02.
- 1 2 3 4 ssc2009 (November 23, 2009). Twin Brown Dwarfs Wrapped in a Blanket. Pasadena, California USA: Caltech. Retrieved 2014-03-12.
- ↑ ESA/PACS/SPIRE/ Consortia (September 1, 2010). Water Around a Carbon Star. Pasadena, California USA: Caltech. Retrieved 2014-03-12.
- ↑ David D. S. Hale; M. Bester; W. C. Danchi; S. Hoss; E. Lipman; J. D. Monnier; P. G. Tuthill; C. H. Townes et al. (20 November 1997). "Multiple dust shells and motions around IK Tauri as seen by infrared interferometry". The Astrophysical Journal 490 (1): 407-411. doi:10.1086/512794. https://pdfs.semanticscholar.org/eaed/d4ec9210c956ad92d66f06be91137db7b749.pdf. Retrieved 2017-07-27.
- 1 2 3 4 5 J. Rho (January 12, 2005). Spitzer/IRAC View of the Trifid Nebula. Pasadena, California USA: NASA/JPL/Caltech. Retrieved 2014-03-06.
- ↑ Howard McCallon (1990). Orion Visible and Infrared. Pasadena, California USA: NASA/JPL. Retrieved 2017-07-21.
- ↑ "The Interstellar Medium". Archived from the original on April 19, 2015. Retrieved May 2, 2015.
- ↑ Panuzzo, P.; Vega, O.; Bressan, A.; Buson, L. et al. (2007). "The Star Formation History of the Virgo Early-Type Galaxy NGC 4435: The Spitzer Mid-Infrared View". The Astrophysical Journal 656 (1): 206–216. doi:10.1086/510147.
- ↑ The Tail of NGC 4435
- ↑ Cortese, L.; Bendo, G. J.; Isaak, K. G.; Davies, J. I. et al. (2010). "Diffuse far-infrared and ultraviolet emission in the NGC 4435/4438 system: tidal stream or Galactic cirrus?". Monthly Notices of the Royal Astronomical Society: Letters 403 (1): L26–L30. doi:10.1111/j.1745-3933.2009.00808.x.
- 1 2 ESA; the PACS Consortium (26 June 2009). Herschels Daring Test: A Glimpse of Things to Come. NASA. Retrieved 2017-07-21.
- ↑ Adolf N. Witt; Karl D. Gordon; Douglas G. Furton (July 1, 1998). "Silicon Nanoparticles: Source of Extended Red Emission?". The Astrophysical Journal Letters 501 (1): L111-5. doi:10.1086/311453. http://iopscience.iop.org/1538-4357/501/1/L111. Retrieved 2013-07-30.
- 1 2 3 4 5 6 J. Gallagher; M. Mountain; P. Puxley (April 24, 2006). Happy Sweet Sixteen, Hubble Telescope!. Baltimore, Maryland USA: HubbleSite.org. Retrieved 2013-07-30.
- ↑ T. Wang; C. Schreiber; D. Elbaz; Y. Yoshimura; K. Kohno; X. Shu; Y. Yamaguchi; M. Pannella et al. (8 August 2019). "A dominant population of optically invisible massive galaxies in the early Universe". Nature Letters 572 (1452): 211-14. doi:10.1038/s41586-019-1452-4. https://www.nature.com/articles/s41586-019-1452-4.epdf?referrer_access_token=ly3DoECoasmJSGIbuvr6ONRgN0jAjWel9jnR3ZoTv0McTGoPc7_FoP-XNr3dFjBSdd_sfbj28wzUtooPhpwug25SJ_NFYLfEOZHG9D-KaO8ab5ehBsQw_UXZGw7HyyN33WADb2Uxv-W1NMGV7GWVFEZSwd82Ahb5EujTlVfR8NJuC-joBREial3N5iSnNgVThmfJ5AbamWAjBWjTTyNXd2SAh6e7drERlwLey-t4HyKGkoicOsjZS9iUN1KD99b4sS9JXEn4fUsVDGOJcMh1sGI8EfpM8erAzLlvL8lmXA_H2LNT1npr_eB-IQU2IwQ5DK15yg_gUiIKKRbtAdkx0sHnSVTsLQpv4nEY8eGIWEQCevYK-hsP9t07ndCGmqsFLGFPWnqY9pCOaeAnSPJSVA%3D%3D&tracking_referrer=www.sciencenews.org. Retrieved 11 August 2019.
- ↑ BLAST Public Webpage
- ↑ SPACE.com (December 25, 2012). NASA Launches Telescope-Toting Balloon from Antarctica on Christmas. SPACE.com. Retrieved 2012-12-26.
- ↑ Govert Schilling (December 26, 2012). NASA Launches Telescope-Toting Balloon from Antarctica on Christmas. McMurdo Station: SPACE.com. Retrieved 2012-12-26.
External links
{{Principles of radiation astronomy}}