
Hydrogens is a lecture on the general nature and specific characteristics of various natural and hominin-made hydrogens. It is an offering from the school of chemistry.
Theoretical hydrogens
Def. any "specific [...] element [such as hydrogen]"[1] is called a hydrogen.
Emissions
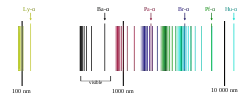
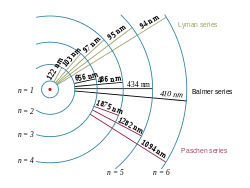
"The emission spectrum of atomic hydrogen is divided into a number of spectral series, with wavelengths given by the Rydberg formula. These observed spectral lines are due to electrons moving between energy levels in the atom. The spectral series are important in astronomy for detecting the presence of hydrogen and calculating red shifts. ... [T]he spectral lines of hydrogen correspond to particular jumps of the electron between energy levels. The simplest model of the hydrogen atom is given by the Bohr model. When an electron jumps from a higher energy to a lower, a photon of a specific wavelength is emitted."[2]
The spectral lines are grouped into series according to n'. Lines are named sequentially starting from the longest wavelength/lowest frequency of the series, using Greek letters within each series. For example, the 2 → 1 line is called "Lyman-alpha" (Ly-α), while the 7 → 3 line is called "Paschen-delta" (Pa-δ). Some hydrogen spectral lines fall outside these series, such as the 21 cm line; these correspond to much rarer atomic events such as hyperfine transitions.[3] The fine structure also results in single spectral lines appearing as two or more closely grouped thinner lines, due to relativistic corrections.[4]
The energy differences between levels in the Bohr model, and hence the wavelengths of emitted/absorbed photons, is given by the Rydberg formula[5]:
where n is the initial energy level, n′ is the final energy level, and R is the Rydberg constant. Meaningful values are returned only when n is greater than n′ and the limit of one over infinity is taken to be zero.
"The familiar red H-alpha [Hα 656 nm] spectral line of hydrogen gas, which is the transition from the shell n = 3 to the Balmer series shell n = 2, is one of the conspicuous colors of the universe. It contributes a bright red line to the spectra of emission or ionization nebula, like the Orion Nebula, which are often H II regions found in star forming regions. In true-color pictures, these nebula have a distinctly pink color from the combination of visible Balmer lines that hydrogen emits."[6]
A hydrogen-alpha filter is an optical filter designed to transmit a narrow bandwidth of light generally centered on the H-alpha wavelength. They are characterized by a bandpass width that measures the width of the wavelength band that is transmitted.[7] These filters are manufactured by multiple (~50) layers of vacuum-deposited layers. These layers are selected to produce interference effects that filter out any wavelengths except at the requisite band.[8] Alternatively, an etalon may be used as the narrow band filter (in conjunction with a "blocking filter" or energy rejection filter) to pass only a narrow (<0.1 nm) range of wavelengths of light centred around the H-alpha emission line. The physics of the etalon and the dichroic interference filters are essentially the same (relying on constructive/destructive interference of light reflecting between surfaces), but the implementation is different (an interference filter relies on the interference of internal reflections). Due to the high velocities sometimes associated with features visible in H-alpha light (such as fast moving prominences and ejections), solar H-alpha etalons can often be tuned (by tilting or changing the temperature) to cope with the associated Doppler effect."[9]
The Balmer series of emission lines from hydrogen occur in the visible spectrum of the Sun at: 397, 410, 434, 486, and 656 nm.
Hydrogen has two emission lines that occur in an electron cyclotron resonance (ECR) heated plasmas at 397.007 nm of the Balmer series (Hε) and 434.05 nm Hγ.[10]
Molecular clouds
"The motions of hydrogen clouds and globular clusters offer probes for the gravitational potential in the outlying parts of massive galaxies; these data also suggest the presence of unseen mass."[11]
"Even if gaseous bound systems of galactic mass can form, the types of galaxy they develop into may depend sensitively on the composition of the gas—in particular, whether it contains heavy elements, which permit more efficient cooling (and whether molecular hydrogen, an important coolant at T < 104K, can form)."[11]
Neutrals
The "efforts to study the 21 cm hydrogen line from the northern and southern hemispheres in 1954 and 1959 [...] were combined and provided the first full-galaxy radio map of neutral hydrogen in the Milky Way".[12]
A hydrogen atom is about 0.11 nm in diameter.
The "relative motion in a hydrogen atom in crossed electric and magnetic fields leads to peculiar quasi-ionized states with an electron localized very far from a proton."[13]
Subatomics
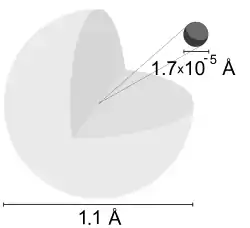
Def. the "lightest and most common isotope of hydrogen, having a single proton and no neutrons- 1
1H"[14] is called protium.
Def. an "isotope of hydrogen formed of one proton and one neutron in each atom - 2
1H"[15] is called deuterium.
"Heavy water is “heavy” because it contains deuterium."[15]
"There were about 80 deuteriums for every million protiums, and virtually no tritium."[15]
Def. a "radioactive isotope of the element hydrogen, (symbol T or 3
1H), having one proton and two neutrons"[16] is called tritium.
Def. a "highly unstable, synthetic isotope of the element hydrogen, 4
1H, having one proton and three neutrons"[17] is called quadrium.
1
1H(p,β+ν)2
1H
At 10-million-kelvin, hydrogen fuses to form helium in the proton-proton chain reaction:[18]
- 41
1H → 22
1H + 2e+ + 2νe (4.0 MeV + 1.0 MeV) - 21
1H + 22
1H → 23
2He + 2γ (5.5 MeV) - 23
2He → 4
2He + 21
1H (12.9 MeV)
These reactions result in the overall reaction:
- 41
1H → 4
2He + 2e+ + 2γ + 2νe (26.7 MeV)
where e+ is a positron, γ is a gamma ray photon, νe is a neutrino, and H and He are isotopes of hydrogen and helium, respectively. The energy released by this reaction is in millions of electron volts, which is actually only a tiny amount of energy.
"The light elements deuterium, lithium, beryllium, and boron pose a special problem for any theory of the origin of the elements which proposes that all the elements are built up from hydrogen in the stars. ... The difficulty arises because the lifetimes of these elements against proton capture, at the temperatures and pressures at which most stellar matter exists, are short compared to the stable lifetimes of stars. These elements then cannot be produced in stellar interiors unless they are transported rapidly to the surface, and if they are produced at the surface, non-equilibrium processes must be involved. Further, they can exist in significant quantities at the surface only in the absence of rapid mixing to the interior."[19]
Muons
Muonium is an exotic atom made up of an antimuon and an electron[20]
During the muon's 2.2 µs lifetime, muonium can enter into compounds such as muonium chloride (MuCl) or sodium muonide (NaMu).[21] Due to the mass difference between the antimuon and the electron, muonium () is more similar to atomic hydrogen (p+e-) than positronium] (e+e-). Its Bohr radius and ionization energy are within 0.5% of hydrogen, deuterium, and tritium.[22]
"Although muonium is short-lived, physical chemists use it in a modified form of electron spin resonance spectroscopy for the analysis of chemical transformations and the structure of compounds with novel or potentially valuable electronic properties. (This form of electron spin resonance (eSR) is called muon spin resonance (μSR).)"[23]
"There are variants of μSR, e.g. muon spin rotation, which is affected by the presence of a magnetic field applied transverse to the muon beam direction (since muons are typically produced in a spin-polarized state from the decay of pions), and avoided level crossing (ALC), which is also called level crossing resonance (LCR)."[23]
"The latter employs a magnetic field applied longitudinally to the beam direction, and monitors the relaxation of muon spins caused by magnetic oscillations with another magnetic nucleus."[23]
Because the muon is a lepton, the atomic energy levels of muonium can be calculated with great precision from quantum electrodynamics (QED), unlike the case of hydrogen, where the precision is limited by uncertainies related to the internal structure of the proton. For this reason, muonium is an ideal system for studying bound-state QED and also for searching for physics beyond the standard model.[24]
Neutrinos
Cosmic "rays interact with the Earth’s atmosphere [109, 110] and with the hydrogen concentrated in the galactic plane [46, 47, 111, 112, 113] producing high-energy neutrinos."[25]
X-rays
"Single ionization and double ionization of elements heavier than helium have only a small effect on the magnitude of X-ray absorption cross sections."[26]
For hydrogen, complete ionization "obviously reduces its cross section to zero, but ... the net effect of partial ionization of hydrogen on calculated absorption depends on whether or not observations of hydrogen [are] used to estimate the total gas. ... [A]t least 20 % of interstellar hydrogen at high galactic latitudes seems to be ionized".[26]
Ultraviolets
"[T]he Lyman series is the series of transitions and resulting ultraviolet emission lines of the hydrogen atom as an electron goes from n ≥ 2 to n = 1 (where n is the principal quantum number referring to the energy level of the electron)."[27]
"The version of the Rydberg formula that generated the Lyman series was[28]:
Where n is a natural number greater than or equal to 2 (i.e. n = 2,3,4,...).
Therefore, the lines seen in the image above are the wavelengths corresponding to on the right, to on the left (there are infinitely many spectral lines, but they become very dense as they approach to (Lyman limit), so only some of the first lines and the last one appear).
The wavelengths (nm) in the Lyman series are all ultraviolet:"[27]
2 | 3 | 4 | 5 | 6 | 7 | 8 | 9 | 10 | 11 | ||
Wavelength (nm) | 121.6 | 102.6 | 97.3 | 95.0 | 93.8 | 93.1 | 92.6 | 92.3 | 92.1 | 91.9 | 91.18 (Lyman limit) |
"In 1913, when Niels Bohr produced his Bohr model theory, the reason why hydrogen spectral lines fit Rydberg's formula was explained. Bohr found that the electron bound to the hydrogen atom must have quantized energy levels described by the following formula:
According to Bohr's third assumption, whenever an electron falls from an initial energy level() to a final energy level(), the atom must emit radiation with a wavelength of:
There is also a more comfortable notation when dealing with energy in units of electronvolts and wavelengths in units of angstroms:
Replacing the energy in the above formula with the expression for the energy in the hydrogen atom where the initial energy corresponds to energy level n and the final energy corresponds to energy level m:
where R_H is the same Rydberg constant for hydrogen of Rydberg's long known formula.
For the connection between Bohr, Rydberg, and Lyman, one must replace m by 1 to obtain:
which is Rydberg's formula for the Lyman series. Therefore, each wavelength of the emission lines corresponds to an electron dropping from a certain energy level (greater than 1) to the first energy level."[27]
Visuals
The Balmer series of emission lines from hydrogen occur in the visible spectrum of the Sun at: 397, 410, 434, 486, and 656 nm. Hα is the red line at the far right in the spectrum at the page top.
Violets
Hydrogen has two emission lines that occur in an electron cyclotron resonance (ECR) heated plasmas at 397.007 nm of the Balmer series (Hε) and 434.05 nm Hγ.[10]
Microwaves
“The hydrogen line, 21 centimeter line or HI line refers to the electromagnetic radiation spectral line that is created by a change in the energy state of neutral hydrogen atoms. This electromagnetic radiation is at the precise frequency of 1420.40575177 [megahertz] MHz, which is equivalent to the vacuum wavelength of 21.10611405413 cm in free space. This wavelength or frequency falls within the microwave radio region of the electromagnetic spectrum, and it is observed frequently in radio astronomy, since those radio waves can penetrate the large clouds of interstellar cosmic dust that are opaque to visible light.”[29]
Plasma objects
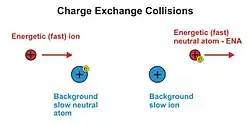
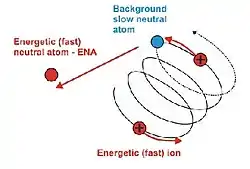
"In 1951, prior to the Space Age, the existence of energetic neutral hydrogen atoms (as high as 70 keV in energy) in space plasma was discovered."[31]
"ENA imaging permits study of the ways in which our entire plasma environment -- including the magnetopause, ring current, plasmasphere, auroral zones, plasma sheet, and the ionosphere -- reacts to the changing conditions of the solar wind (Williams, 1990)."[31]
"Proton–hydrogen charge-exchange collisions [such as those shown at right] are often the most important process in space plasma because hydrogen is the most abundant constituent of both plasmas and background gases and hydrogen charge-exchange occurs at very high velocities involving little exchange of momentum."[32]
Nucleosynthesis
1H(p,β+ν)2H
At "10-million-kelvin ..., hydrogen fuses to form helium in the proton-proton chain reaction:[18]
- 41H → 22H + 2e+ + 2νe (4.0 MeV + 1.0 MeV)
- 21H + 22H → 23He + 2γ (5.5 MeV)
- 23He → 4He + 21H (12.9 MeV)"[33]
"These reactions result in the overall reaction:
- 41H → 4He + 2e+ + 2γ + 2νe (26.7 MeV)
where e+ is a positron, γ is a gamma ray photon, νe is a neutrino, and H and He are isotopes of hydrogen and helium, respectively. The energy released by this reaction is in millions of electron volts, which is actually only a tiny amount of energy."[33]
"The light elements deuterium, lithium, beryllium, and boron pose a special problem for any theory of the origin of the elements which proposes that all the elements are built up from hydrogen in the stars. ... The difficulty arises because the lifetimes of these elements against proton capture, at the temperatures and pressures at which most stellar matter exists, are short compared to the stable lifetimes of stars. These elements then cannot be produced in stellar interiors unless they are transported rapidly to the surface, and if they are produced at the surface, non-equilibrium processes must be involved. Further, they can exist in significant quantities at the surface only in the absence of rapid mixing to the interior."[19]
Gases
Hydrogen can exist as a diatomic gas (H2) or a monatomic gas. Each hydrogen isotope (hydrogen, deuterium, or tritium) can exist in each form of the gas.
Diatomic gases
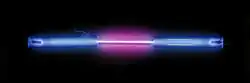
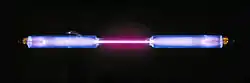
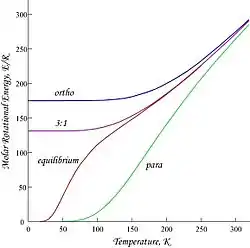
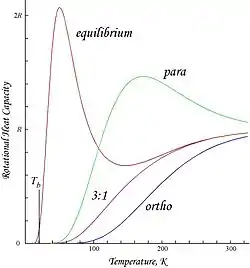
Molecular hydrogen gas is excited in the discharge tube shown on the right. When an electron returns to a lower energy orbital state the purple color is observed.
"Molecular hydrogen (H2) [is] a colourless, odourless and flammable gas at room temperature."[34]
"The familiar red H-alpha [Hα 656 nm] spectral line of hydrogen gas, which is the transition from the shell n = 3 to the Balmer series shell n = 2, is one of the conspicuous colors of the universe. It contributes a bright red line to the spectra of emission or ionization nebula, like the Orion Nebula, which are often H II regions found in star forming regions. In true-color pictures, these nebula have a distinctly pink color from the combination of visible Balmer lines that hydrogen emits."[6]
A "high-resolution spectrum of the Becklin-Neugebauer (BN) infrared point source located in [the region of the Orion Nebula] ... with the Steward Observatory 2.29 m (90 inch) telescope ... [confirmed] the reality of [the 2.12 μ] line ... on 1976 January 15 and 16. The line was then identified by R. Treffers as the S(1) line of the 1-0 vibration-rotation quadrupole spectrum of H2. Six other lines of the same band were also found. The presence of two of our lines has been confirmed by Grasdalen and Joyce (1976). Electronic transitions of interstellar H2 have previously been observed in the ultraviolet (Carruthers 1970; Smith 1973; Spitzer et al. 1973)."[35]
Diatomic hydrogen gas apparently exists in two distinct forms which can mix: orthohydrogen and parahydrogen. On the right is a graph of molar energies of orthohydrogen and parahydrogen, plus an equilibrium mixture obtained when a catalyst is present to allow for ortho-para interconversion. The curve marked 3:1 is the ortho:para ratio at room temperature that will persist if no catalyst is present during cooling. Orthohydrogen has the spins of the two protons parallel while para hydrogen has them antiparallel.
The second graph shows the molar heat capacities for the same gases versus temperature.
Monatomic gases
As temperature increases in an astronomical object composed of H2, the molecules begin to dissociate.
"At a temperature of 8000 K, hydrogen gas is 99.99 percent monatomic."[36]
where is an initial concentration [H] at low temperatures as partial particle density, is the dissociation energy 4.52 eV, k is Boltzmann's contant (8.6173324(78)×10−5 eV K-1), and T is temperature in K.
Liquids

The image on the right shows that liquid hydrogen can be poured.
Solids
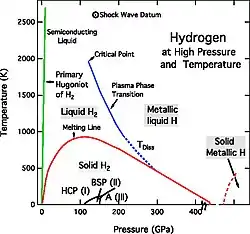
"Slush hydrogen [is] a mixture of solid and liquid hydrogen".[37]
Solid hydrogen is achieved by decreasing the temperature below hydrogen's melting point of 14.01 K.[38]
Native solid hydrogen (mineral) is not known to occur on the surface of the Earth. As the pressure-temperature phase (PTP) diagram for hydrogen on the right suggests there can be solid metallic monatomic hydrogen and solid H2 at cryogenic temperatures and pressures, including room pressure or atmospheric pressure.
Solid hydrogen (H2) occurs in a hcp structure transitioning to a broken symmetry phase (BSP), then to the A phase at atmospheric pressure with increasing temperature.
Organics
"1H, the most commonly used spin ½ nucleus in NMR investigation, has been studied using many forms of NMR. Hydrogen is highly abundant, especially in biological systems. It is the nucleus most sensitive to NMR signal (apart from 3H which is not commonly used due to its instability and radioactivity). Proton NMR produces narrow chemical shift with sharp signals. Fast acquisition of quantitative results (peak integrals in stoichiometric ratio) is possible due to short relaxation time."[39]
"[NMR] widely used in chemical studies, notably in NMR spectroscopy such as proton NMR, carbon-13 NMR, deuterium NMR and phosphorus-31 NMR."[39]
"Proton NMR (also Hydrogen-1 NMR, or 1H NMR) is the application of nuclear magnetic resonance in NMR spectroscopy with respect to hydrogen-1 nuclei within the molecules of a substance, in order to determine the structure of its molecules.[40] In samples where natural hydrogen (H) is used, practically all of the hydrogen consists of the isotope 1H (hydrogen-1; i.e. having a proton for a nucleus). A full 1H atom is called protium."[41]
Bio-organics
"Use of an appropriate hydrogen level is necessary to favor dehalogenation of chlorinated solvents, such as tetrachloroethene (PCE) and trichloroethene (TCE), over other hydrogen using processes. ... the competition between dehalogenators and other microorganisms occurring in a benzoate-acclimated dehalogenating methanogenic mixed culture. ... the dehalogenators competed best against methanogens and homoacetogens when the hydrogen level was maintained between 2 and 11 nM."[42]
"In contrast, with hydrogen as the primary electron donor, homoacetogens became the dominant group in hydrogen utilization with their advantageous kinetic properties."[42]
Glaciology
"Isotopic exchange between ice and water is found to take place in temperate glaciers. This exchange causes homogenization of deuterium in snow during summer thaws, together with a general increase in deuterium concentration."[43]
Sun
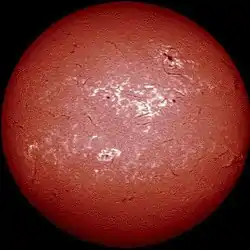
Depending primarily upon gas temperature, the presence of gas may be used to determine the composition of the gas object observed, at least the outer layer. Early spectroscopy[44] of the Sun using estimates of "the line intensities of several lines by eye [to derive] the abundances of ... elements ... [concluded] that the Sun [is] largely made of hydrogen."[45]
The composition in the photosphere of the modern-day Sun, by mass, is 74.9% hydrogen and 23.8% helium.[46]
The visible light we see is produced as electrons react with hydrogen atoms to produce H− ions.[47][48]
"In the 1920s, Payne [3] and Russell [4] reported that the Sun’s atmosphere consisted mostly of hydrogen (H) and helium (He), but Hoyle [5] notes that he and others "in the astronomical circles to which I was privy" (p. 153) continued until after the Second World War to believe that the Sun was made mostly of iron. Then Hoyle notes that "much to my surprise" (p. 154), the high-hydrogen, low-iron model was suddenly adopted without opposition."[49]
For comparison with other stars, the Sun has the following properties:
- The effective temperature of the surface of the Sun's photosphere is 5,778 K.[50]
- Metallicity, Z = 0.0122[51], "lowest seismic estimate of solar metallicity is Z = 0.0187 [to the] highest is Z = 0.0239, with uncertainties in the range of 12%-19%."[52]
- Stellar companion: Jupiter at present, perhaps Uranus in some larger form previously[53]
- Age: 4.57 billion years[54]
- B-V = 0.656 ± 0.005[55]
- Rotation rate: 7.189 x 103 km h-1 (at the equator), equatorial circumference of 4,379,000 kilometres divided by sidereal rotation period of 609.12 hours[56]
Photosphere volumes
R⊙eq ≈ 6.955 x 105 km. The thickness of the photosphere is about 400 km. R⊙p ≈ 6.951 x 105 km.
Photosphere hydrogens
The density of the Sun is about 2 x 10-4 kg m-3. Or,
One mole of H2 (gas) has a mass of 2.016 x 10-3 kg. The molar density of the photosphere may be
Constant volume specific heat capacity
For H2 (gas) the molar constant-volume heat capacity at 298 K is 20.18 J/(mol · K). At 2000 K it is about 25 J/(mol · K). Using a linear extrapolation,
for 5777 K, yields
Before calculating the amount of energy or power necessary to heat the coronal clouds around the Sun, let's see if the influx of electrons from outside the heliosphere may be able to heat the surface of the photosphere (p) to 5777 K from 100 K.
Photosphere heating
Voyager 1 is 17,932,000,000 km (119.9 AU) from the Sun at RA 17.163h Dec +12.44°, ecliptic latitude of 34.9°.
For this laboratory example, let the electron flux be 2 e- cm-2 s-1 diffusing into our solar system from elsewhere in the galaxy. Each of these electrons has an energy of 10 MeV.
If the electron flux measured by Voyager 1 is close to 2 e- cm-2 s-1 where each electron averages 10 MeV and these electrons are heading for the Sun, then each electron may strike the photosphere from anywhere in a sphere around the Sun.
To heat the photosphere to 5777 K takes
The power (P) that may be deposited on the photospheric surface of the Sun is
The luminosity (in Watts, W) of the Sun is 3.846 x 1026 W. In eV/s this is
If the energy of the incoming electrons is 700 MeV and the flux is 8.48 x 104 e- cm-2 s-1, then the power from the incoming electrons would be
Heliognosy
Depending primarily upon gas temperature, the presence of gas may be used to determine the composition of the gas object observed, at least the outer layer. Early spectroscopy[44] of the Sun using estimates of "the line intensities of several lines by eye [to derive] the abundances of ... elements ... [concluded] that the Sun [is] largely made of hydrogen."[45]
As temperature increases in an astronomical object composed of H2 gas, the molecules begin to dissociate.
"At a temperature of 8000 K, hydrogen gas is 99.99 percent monatomic."[36]
where is an initial concentration [H] at low temperatures as partial particle density, is the dissociation energy 4.52 eV, k is Boltzmann's contant (8.6173324(78)×10−5 eV K-1), and T is temperature in K.
Using
- what is the concentration of H ([H]) at T = 8000 K?
- what is [H] at T = 800 K?
- at what temperature is [H] = 1?
- what is [H] at T = 5778 K?
At 5778 K [H] = 8 %.
Earth
"The hydrogen in the sun provides the Earth with abundant renewable solar energy that creates its own cascades of abundance. It is solar energy that drives all living systems, the water cycle, the global circulation of air. Properly tapped, it will provide humanity with inexhaustible sources of solar, wind, water and ocean energy that can make dirtier non-renewable sources like fossil and radioactive fuels unnecessary."[57]
Bacteriology
"Use of an appropriate hydrogen level is necessary to favor dehalogenation of chlorinated solvents, such as tetrachloroethene (PCE) and trichloroethene (TCE), over other hydrogen using processes. ... the competition between dehalogenators and other microorganisms occurring in a benzoate-acclimated dehalogenating methanogenic mixed culture. ... the dehalogenators competed best against methanogens and homoacetogens when the hydrogen level was maintained between 2 and 11 nM."[42]
"In contrast, with hydrogen as the primary electron donor, homoacetogens became the dominant group in hydrogen utilization with their advantageous kinetic properties."[42]
Betelgeuse
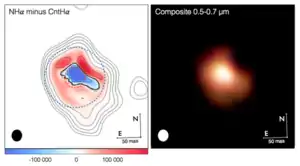
"Two of the filters used to image Betelgeuse are sensitive to the familiar red hydrogen alpha spectral feature. Because one filter is broader than the other, subtracting the light in the narrow filter from the light seen with the broad filter yields a map of where hydrogen gas is emitting or absorbing light. It also turns out to be highly asymmetric. Most of the hydrogen emission is confined within a distance of three times Betelgeuse’s near-infrared radius. It’s a similar distance from the star as most of the polarized dust, but the spatial distributions are different."[58]
In the image on the right, "Left: A map of hydrogen emission (red) and absorption (blue) in the vicinity of Betelgeuse, with the same dashed lines [The red dashed circle indicates Betelgeuse’s infrared photospheric radius. The light dashed circle is three times this.] for reference. Right: Color composite of three of the filters from the first figure (the narrow hydrogen alpha filter is excluded)."[58]
"Betelgeuse’s asymmetries persist in both in dust and gas, with a major interface between the two located around three times the near-infrared stellar radius. These asymmetries agree with different types of past observations and also strongly point toward a connection between supergiant mass loss and vigorous convection."[58]
Interstellar medium
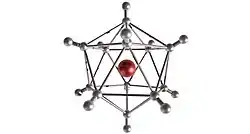
"[O]nce ionized, the gas is rapidly heated by Coulomb collisions to the coronal cloud temperature, but as this material peels off, a cooler hydrogen-emitting region is left."[59]
A number of spectral lines produced by interstellar gas, notably the hydrogen spectral line at 21 cm, are observable at radio wavelengths.[60][61]
"The familiar red H-alpha [Hα 656 nm] spectral line of hydrogen gas, which is the transition from the shell n = 3 to the Balmer series shell n = 2, is one of the conspicuous colors of the universe. It contributes a bright red line to the spectra of emission or ionization nebula, like the Orion Nebula, which are often H II regions found in star forming regions. In true-color pictures, these nebula have a distinctly pink color from the combination of visible Balmer lines that hydrogen emits."[6]
"Hydrogen molecules made up of two hydrogen atoms (pairs of silver spheres) surround a negatively charged hydrogen ion (red sphere) [in the image on the right]. The molecules arrange into an icosahedron, a shape with 12 vertices."[62]
"Each cluster consists of hydrogen molecules arranged around a negatively charged hydrogen ion — a single hydrogen atom with an extra electron — at temperatures near absolute zero."[62]
"Similar, positively charged ion clusters have previously been found."[62]
Infused "tiny droplets of liquid helium with hydrogen gas [were] bombarded [...] with a beam of electrons, which converted some hydrogen molecules into negatively charged hydrogen ions. Neighboring hydrogen molecules (two bonded hydrogen atoms) clustered around the ion, in groups of a few molecules to over 60."[62]
The "geometric structures of the clusters [consisted of] Hydrogen molecules organized into shells that surrounded the central ion. Clusters were most stable, and most common, when molecules filled shells to their capacity. In the first shell, for example, the cluster formed an icosahedron — a 3-D shape with 12 vertices — when 12 molecules filled this shell."[62]
"In space, hydrogen cluster ions might form naturally in cold, dense clouds of hydrogen or in atmospheres of gas giant planets."[62]
Milky Way
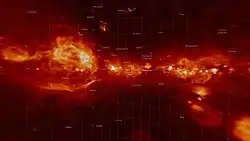
"Spectra of the helium 2.06 µm and hydrogen 2.17 µm lines ... confirm the existence of an extended region of high-velocity redshifted line emission centered near [Sgr A*/IRS 16]."[63]
Recent history
The recent history period dates from around 1,000 b2k to present.
"Hydrogen was discovered in 1766, by Henry Cavendish in London, UK (even though scientists have been producing hydrogen for years ... Written records said that chemist Robert Boyle has been producing hydrogen as early as 1671!). Antoine Lavoisier gave the element its name, derived from the Greek 'hydro' (ὑδρο) and 'genes' (γενής) , meaning water-forming."[64]
Radiation astromathematics
"The energy differences between levels in the Bohr model, and hence the wavelengths of emitted/absorbed photons, is given by the Rydberg formula[5]:
where n is the initial energy level, n′ is the final energy level, and R is the Rydberg constant. Meaningful values are returned only when n is greater than n′ and the limit of one over infinity is taken to be zero."[2]
Technology
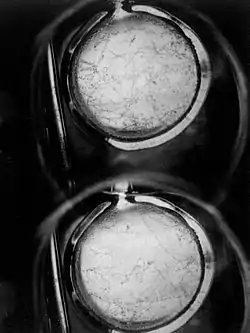

The first image on the left shows a liquid hydrogen bubble chamber used to detect subatomic particles from a bevatron.
On the right, the second image down, a Space Shuttle Main Engine burns diatomic hydrogen from the liquid hydrogen fuel tank with diatomic oxygen from the liquid oxygen fuel tank, producing a nearly invisible flame at full thrust.
Chemically,
See also
References
- ↑ chemical. San Francisco, California: Wikimedia Foundation, Inc. 9 March 2015. Retrieved 2015-07-16.
- 1 2 "Hydrogen spectral series". San Francisco, California: Wikimedia Foundation, Inc. May 2, 2012. Retrieved 2012-05-14.
- ↑ The Hydrogen 21-cm Line, In: Hyperphysics. Georgia State University. 2004-10-30. Retrieved 2009-03-18.
- ↑ Richard L. Liboff (2002). Introductory Quantum Mechanics. Addison-Wesley. ISBN 0-8053-8714-5.
- 1 2 Niels Bohr (1985), "Rydberg's discovery of the spectral laws", in J. Kalckar (ed.), N. Bohr: Collected Works, 10, Amsterdam: North-Holland Publ., pp. 373–9
- 1 2 3 "Balmer series". San Francisco, California: Wikimedia Foundation, Inc. February 2, 2012. Retrieved 2012-07-11.
- ↑ "Filters". Astro-Tom.com. Retrieved 2006-12-09.
- ↑ D. B. Murphy; K. R. Spring; M. J. Parry-Hill; I. D. Johnson; M. W. Davidson. "Interference Filters". Olympus. Retrieved 2006-12-09.
- ↑ "H-alpha". San Francisco, California: Wikimedia Foundation, Inc. December 30, 2011. Retrieved 2012-07-11.
- 1 2 K. J. McCarthy; A. Baciero; B. Zurro; TJ-II Team (12 June 2000). Impurity Behaviour Studies in the TJ-II Stellarator, In: 27th EPS Conference on Contr. Fusion and Plasma Phys. (PDF). 24B. Budapest: ECA. pp. 1244–7. Retrieved 2013-01-20.
- 1 2 Martin J. Rees (December 1984). "Is the Universe flat?". Journal of Astrophysics and Astronomy 5 (4): 331-48. http://link.springer.com/article/10.1007/BF02714464. Retrieved 2013-12-18.
- ↑ Whitham D. Reeve (1973). "Book Review" (PDF). Anchorage, Alaska USA: Whitham D. Reeve. Retrieved 2014-01-11.
- ↑ I. Dzyaloshinskii (May 1992). "Effects of the finite proton mass in a hydrogen atom in crossed magnetic and electric fields: a state with a giant electric dipole moment". Physics Letters A 165 (1): 69-71. doi:10.1016/0375-9601(92)91056-W. http://www.sciencedirect.com/science/article/pii/037596019291056W. Retrieved 2014-02-13.
- ↑ SemperBlotto (12 November 2005). "protium". San Francisco, California: Wikimedia Foundation, Inc. Retrieved 2015-07-20.
- 1 2 3 "deuterium". San Francisco, California: Wikimedia Foundation, Inc. 16 July 2015. Retrieved 2015-07-20.
- ↑ "tritium". San Francisco, California: Wikimedia Foundation, Inc. 16 July 2015. Retrieved 2015-07-20.
- ↑ SemperBlotto (2 June 2012). "quadrium". San Francisco, California: Wikimedia Foundation, Inc. Retrieved 2015-07-20.
- 1 2 G. Wallerstein; I. Iben Jr.; P. Parker; A. M. Boesgaard; G. M. Hale; A. E. Champagne; C. A. Barnes; F. KM-dppeler et al. (1999). "Synthesis of the elements in stars: forty years of progress". Reviews of Modern Physics 69 (4): 995–1084. doi:10.1103/RevModPhys.69.995. http://authors.library.caltech.edu/10255/1/WALrmp97.pdf. Retrieved 2006-08-04.
- 1 2 Walter K. Bonsack (November 1959). "The Abundance of Lithium and Convective Mixing in Stars of Type K". The Astrophysical Journal 130 (11): 843-71. doi:10.1086/146777.
- ↑ International Union of Pure and Applied Chemistry (IUPAC) (1997). A.D. McNaught; A. Wilkinson (eds.). Muonium, In: Compendium of Chemical Terminology (2nd ed.). Blackwell Scientific Publications. doi:10.1351/goldbook.M04069. ISBN 0-86542-684-8.
- ↑ W.H. Koppenol (International Union of Pure and Applied Chemistry) (2001). "Names for muonium and hydrogen atoms and their ions". Pure and Applied Chemistry 73 (2): 377–380. doi:10.1351/pac200173020377. http://www.iupac.org/publications/pac/2001/pdf/7302x0377.pdf.
- ↑ Walker, David C (1983-09-08). Muon and Muonium Chemistry. p. 4. ISBN 978-0-521-24241-7.
- 1 2 3 "Muonium". San Francisco, California: Wikimedia Foundation, Inc. November 14, 2013. Retrieved 2014-03-23.
- ↑ K.P. Jungmann (2004). "Past, Present and Future of Muonium". Proc. of Memorial Symp. in Honor of V. W. Hughes, New Haven, Connecticut, 14–15 Nov 2003: 134. doi:10.1142/9789812702425_0009. ISBN 978-981-256-050-6.
- ↑ Francis Halzen; Dan Hooper (June 12, 2002). "High-energy neutrino astronomy: the cosmic ray connection". Reports on Progress in Physics 65 (7): 1025-107. doi:10.1088/0034-4885/65/7/201. http://iopscience.iop.org/0034-4885/65/7/201. Retrieved 2014-02-08.
- 1 2 Robert Morrison; Dan McCammon (July 1983). "Interstellar photoelectric absorption cross sections, 0.03-10 keV". The Astrophysical Journal 270 (7): 119-22.
- 1 2 3 "Lyman series". San Francisco, California: Wikimedia Foundation, Inc. April 19, 2012. Retrieved 2012-06-26.
- ↑ John Brehm and William Mullin, Introduction to the Structure of Matter, John Wiley & Sons, Inc., 1989, p. 156 ISBN 0-471-60531-X
- ↑ "Hydrogen line". San Francisco, California: Wikimedia Foundation, Inc. August 23, 2012. Retrieved 2012-08-29.
- 1 2 Mike Gruntman. "Charge Exchange Diagrams". Energetic Neutral Atoms Tutorial. Retrieved 2009-10-27.
- 1 2 K. C. Hsieh; C. C. Curtis (1998). Imaging Space Plasma With Energetic Neutral Atoms Without Ionization, In: Measurement Techniques in Space Plasmas: Fields. Geophysical Monograph 103. American Geophysical Union. pp. 235-49. http://www.agu.org/books/gm/v103/GM103p0235/GM103p0235.pdf. Retrieved 2014-10-02.
- ↑ "Energetic neutral atom". San Francisco, California: Wikimedia Foundation, Inc. August 4, 2012. Retrieved 2012-08-12.
- 1 2 "Star". San Francisco, California: Wikimedia Foundation, Inc. July 13, 2013. Retrieved 2013-07-12.
- ↑ "hydrogen". San Francisco, California: Wikimedia Foundation, Inc. September 1, 2013. Retrieved 2013-10-05.
- ↑ T. N. Gautier II; Uwe Fink; Richard R. Treffers; Harold P. Larson (July 15, 1976). "Detection of Molecular Hydrogen Quadrupole Emission in the Orion Nebula". The Astrophysical Journal 207 (07): L129-33. doi:10.1086/182195. http://adsabs.harvard.edu/full/1976ApJ...207L.129G. Retrieved 2013-10-05.
- 1 2 Paul A. Tipler; Gene Mosca (May 1, 2007). Physics for Scientists and Engineers. Macmillan. p. 1172. ISBN 142920124X. Retrieved 2014-01-02.
- ↑ Nancy B. McNelis; Terry L. Hardy; Margaret V. Whalen; Maureen T. Kudlac; Matthew E. Moran; Thomas M. Tomsik; Mark S. Haberbusch (3 April 1995). "A Summary of the Slush Hydrogen Technology Program for the National Aero-Space Plane". Cleveland, Ohio USA: National Aeronautics and Space Administration. Retrieved 2015-07-20.
- ↑ James Dewar (1899). "Sur la solidification de l'hydrogène". Annales de Chimie et de Physique 18: 145–150. http://gallica.bnf.fr/ark:/12148/bpt6k349183/f143.table.
- 1 2 "Nuclear magnetic resonance". San Francisco, California: Wikimedia Foundation, Inc. October 31, 2014. Retrieved 2014-01-22.
- ↑ R. M. Silverstein, G. C. Bassler and T. C. Morrill, Spectrometric Identification of Organic Compounds, 5th Ed., Wiley, 1991.
- ↑ "Proton NMR". San Francisco, California: Wikimedia Foundation, Inc. January 23, 2014. Retrieved 2014-01-22.
- 1 2 3 4 Yanru Yang; Perry L. McCarty (September 26, 1998). "Competition for Hydrogen within a Chlorinated Solvent Dehalogenating Anaerobic Mixed Culture". Environmental Science & Technology 32 (22): 3591-7. doi:10.1021/es980363n. http://lib3.dss.go.th/fulltext/Journal/Environ%20Sci.%20Technology1998-2001/1998/no.22/22,1998%20vol.32,no.22,p3591-3597.pdf. Retrieved 2012-03-16.
- ↑ Bragi Árnason (1969). "The exchange of hydrogen isotopes between ice and water in temperature glaciers". Earth and Planetary Science Letters 6 (6): 423-30. doi:10.1016/0012-821X(69)90111-3. http://adsabs.harvard.edu/abs/1969E%26PSL...6..423A. Retrieved 2014-09-20.
- 1 2 H. N. Russell (1929). The Astrophysical Journal 70: 11-82.
- 1 2 Sarbani Basu; H. M. Antia (March 2008). "Helioseismology and Solar Abundances". Physics Reports 457 (5-6): 217-83. doi:10.1016/j.physrep.2007.12.002.
- ↑ Lodders, K. (2003). "Abundances and Condensation Temperatures of the Elements". Meteoritics & Planetary Science 38 (suppl.): 5272. doi:10.1086/375492. http://www.lpi.usra.edu/meetings/metsoc2003/pdf/5272.pdf.
- ↑ E.G. Gibson (1973). The Quiet Sun. NASA. ASIN B0006C7RS0.
- ↑ Shu, F.H. (1991). The Physics of Astrophysics. 1. University Science Books. ISBN 0-935702-64-4.
- ↑ O. Manuel; C. Bolon; A. Katragada; M. Insall (2001). "Attraction and Repulsion of Nucleons: Sources of Stellar Energy". Journal of Fusion Energy 19 (1): 93-8. doi:10.1023/A:1012290028638. http://link.springer.com/article/10.1023/A:1012290028638. Retrieved 2014-04-13.
- ↑ David R. Williams (September 2004). "Sun Fact Sheet". Greenbelt, MD: NASA Goddard Space Flight Center. Retrieved 2011-12-20.
- ↑ M. Asplund; N. Grevesse; A. J. Sauval (January 2006). "The new solar abundances - Part I: the observations". Communications in Asteroseismology 147 (01): 76-9. doi:10.1553/cia147s76. http://adsabs.harvard.edu/abs/2006CoAst.147...76A. Retrieved 2013-08-08.
- ↑ William J. Chaplin; Aldo M. Serenelli; Sarbani Basu; Yvonne Elsworth; Roger New; Graham A. Verner (November 20, 2007). "Solar Heavy-Element Abundance: Constraints from Frequency Separation Ratios of Low-Degree p-Modes". The Astrophysical Journal 670 (1): 872-84. doi:10.1086/522578. http://adsabs.harvard.edu/abs/2007ApJ...670..872C. Retrieved 2013-08-08.
- ↑ "Uranus". San Francisco, California: Wikimedia Foundation, Inc. December 18, 2012. Retrieved 2012-12-23.
- ↑ A. Bonanno; H. Schlattl; L. Paternò (2008). "The age of the Sun and the relativistic corrections in the EOS". Astronomy and Astrophysics 390 (3): 1115–1118. doi:10.1051/0004-6361:20020749.
- ↑ David F. Gray (November 1992). "The Inferred Color Index of the Sun". Publications of the Astronomical Society of the Pacific 104 (681): 1035-8.
- ↑ Samantha Harvey (April 26, 2007). "Solar System Exploration". Washington, DC USA: National Aeronautics and Space Administration. Retrieved 2013-08-08.
- ↑ Roberto Verzola (October 2009). "21st-Century Political Economies: Beyond Information Abundance". Ethics of Waste in the Information Society 11 (10): 52-61. http://scidok.sulb.uni-saarland.de/volltexte/2009/2536/pdf/irie_11.pdf#page=54. Retrieved 2014-07-20.
- 1 2 3 Meredith Rawls (24 November 2015). "Zooming in on Betelgeuse". Astrobites. Retrieved 2016-01-01.
- ↑ Harold Zirin (June 1978). "The L-alpha/H-alpha ratio in solar flares, quasars, and the chromosphere". Astrophysical Journal 222 (6): L105-7. doi:10.1086/182702.
- ↑ F. H. Shu (1982). The Physical Universe. Mill Valley, California: University Science Books. ISBN 0-935702-05-9.
- ↑ Cox, A. N., ed. (2000). Allen's Astrophysical Quantities. New York: Springer-Verlag. p. 124. ISBN 0-387-98746-0.
- 1 2 3 4 5 6 Emily Conover (9 January 2017). "New form of hydrogen created, Negatively charged clusters might exist in outer space". Science News. Retrieved 2017-01-11.
- ↑ T. R. Geballe; K. Krisciunas; J. A. Bailey; R. Wade (April 1, 1991). "Mapping of infrared helium and hydrogen line profiles in the central few arcseconds of the Galaxy". The Astrophysical Journal 370 (4): L73-6. doi:10.1086/185980. http://adsabs.harvard.edu/abs/1991ApJ...370L..73G. Retrieved 2012-08-03.
- ↑ "The periodic table/Hydrogen, In: Wikiversity". San Francisco, California: Wikimedia Foundation, Inc. 18 August 2016. Retrieved 2016-08-17.