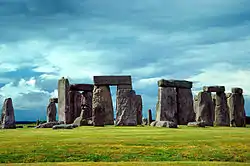
The Hominidae, or hominins have apparently been on Earth for around seven to eight million years, at least somewhere in Africa and elsewhere. Fortunately and deliberately, many of these have worked out ways to record knowledge about the objects or entities in the sky observed by the radiation they produce. Original research/Radiation astronomy as an observational science has a long radiation history.
Archaeoastronomy
Def. (from 1945) "those parts of human interest in celestial phenomena which are amenable to mathematical treatment" are called astronomy.[2] This is Neugebauer's definition of astronomy.[3]
Def. "the search for astronomical strata whose contents could be classified" is called astronomy.[4] This is Herschel's definition of astronomy.[5]
"Mere "star ordering" is not "astronomy", so far as the modern usage of the term implies, regardless of the word's etymology".[6]
Def. (from 2000) "the accurate mapping of the heavens in order to make possible the accurate prediction of phenomena" is called astronomy.[6]
Def. "the study of objects and matter outside the Earth's atmosphere and of their physical and chemical properties" is called astronomy.[7]
"The objective of the [Lunar Dust Environment and Plasma Package (L-DEPP)] activity is to study a package to determine the charging, levitation and transport of lunar dust, in-situ on the Moon, and the associated properties of the local electric fields and plasma environment. The study will [provide inputs], including [observe] radio spectrum (with an additional goal to prepare for future radiation astronomy activities".[8]
"There is nothing in the world that cannot be understood. There are only things that are not yet understood. Two new weapons have appeared recently for the research in astral bodies. One is radiation astronomy; the other is astro-navigation. These will greatly speed up our understanding of the structure of the planets,1 satellites and comets."[9]
Planetary sciences
The history of planetary science may be said to have begun with the Ancient Greek philosopher Democritus, who is reported by Hippolytus as saying
The ordered worlds are boundless and differ in size, and that in some there is neither sun nor moon, but that in others, both are greater than with us, and yet with others more in number. And that the intervals between the ordered worlds are unequal, here more and there less, and that some increase, others flourish and others decay, and here they come into being and there they are eclipsed. But that they are destroyed by colliding with one another. And that some ordered worlds are bare of animals and plants and all water.[10]
Theoretical radiation astrohistory
Def. the study of alien history, from science fiction, is called astrohistory.
"Unlike most astro-history books The History and Practice of Ancient Astronomy is replete with a host of exercises and suggestion for observations."[11]
"It is simply to acknowledge that others have served the genre much better, and that if this work is judged to have any merit it will not be for adding significantly to that splendid astrohistorical collection."[12]
"In one sense, the periodic table is actually irrelevant to studying the astrohistory of the elements. [...] Unfortunately, one sad truth of astrohistory is that Sagan's "star stuff" didn't grace every part of our planet equally."[13]
Here's a theoretical definition:
Def. the astrohistory of the use by hominins of radiation astronomy is called radiation astrohistory.
Entities
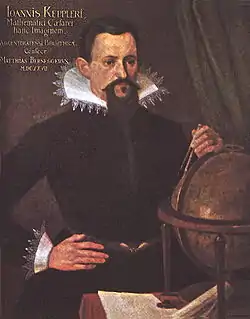
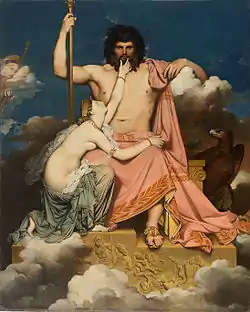
Johannes Kepler is a German mathematician, astronomer and astrologer. A key figure in the 17th century scientific revolution, he is best known for his eponymous laws of planetary motion, codified by later astronomers, based on his works Astronomia nova, Harmonices Mundi, and Epitome of Copernican Astronomy.
With respect to the beginnings of projective geometry, Kepler introduced the idea of continuous change of a mathematical entity in this work. He argued that if a focus of a conic section were allowed to move along the line joining the foci, the geometric form would morph or degenerate, one into another. In this way, an ellipse becomes a parabola when a focus moves toward infinity, and when two foci of an ellipse merge into one another, a circle is formed. As the foci of a hyperbola merge into one another, the hyperbola becomes a pair of straight lines. He also assumed that if a straight line is extended to infinity it will meet itself at a single point at infinity, thus having the properties of a large circle.[14]
Many mythologies have deities especially associated with the sky. The Egyptian god Horus was the god of the Sky. Zeus was the god of the sky and thunder in Greek mythology. The Roman god Jupiter was the god of sky and thunder. Many cultures have drawn constellations between stars in the sky, using them in association with legends and mythology about their deities.
Uranus ... Ouranos meaning "sky" or "heaven") was the primal Greek god personifying the sky. His equivalent in Roman mythology was Caelus. In Ancient Greek literature, Uranus or Father Sky was the son and husband of Gaia, Mother Earth.
Cosmic rays
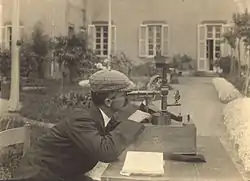
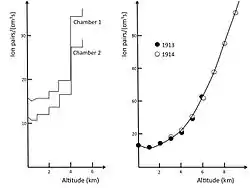
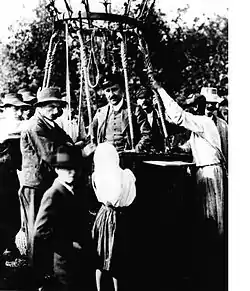
In 1909 Theodor Wulf developed an electrometer, a device to measure the rate of ion production inside a hermetically sealed container, and used it to show higher levels of radiation at the top of the Eiffel Tower than at its base. However, his paper published in Physikalische Zeitschrift was not widely accepted. In 1911 Domenico Pacini observed simultaneous variations of the rate of ionization over a lake, over the sea, and at a depth of 3 meters from the surface. Pacini concluded from the decrease of radioactivity underwater that a certain part of the ionization must be due to sources other than the radioactivity of the Earth.[15]
Then, in 1912, Victor Hess carried three enhanced-accuracy Wulf electrometers[16] to an altitude of 5300 meters in a free balloon flight. He found the ionization rate increased approximately fourfold over the rate at ground level.[16] Hess also ruled out the Sun as the radiation's source by making a balloon ascent during a near-total eclipse. With the moon blocking much of the Sun's visible radiation, Hess still measured rising radiation at rising altitudes.[16] He concluded "The results of my observation are best explained by the assumption that a radiation of very great penetrating power enters our atmosphere from above." In 1913–1914, Werner Kolhörster confirmed Victor Hess' earlier results by measuring the increased ionization rate at an altitude of 9 km. Hess received the Nobel Prize in Physics in 1936 for his discovery.[17][18]
The Hess balloon flight took place on 07 August 1912, providing the first direct evidence of cosmic radiation.
Neutrons
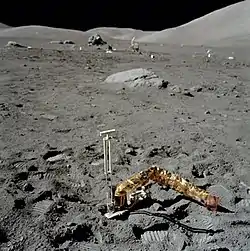
In 1920, Ernest Rutherford conceived the possible existence of the neutron.[19] In particular, Rutherford considered that the disparity found between the atomic number of an atom and its atomic mass could be explained by the existence of a neutrally charged particle within the atomic nucleus. He considered the neutron to be a neutral double consisting of an electron orbiting a proton.[20]
"In 1930 Viktor Ambartsumian and Dmitri Ivanenko in USSR found that, contrary to the prevailing opinion of the time, the nucleus cannot consist of protons and electrons. They proved that some neutral particles must be present besides the protons.[21][22]
In 1931, Walther Bothe and Herbert Becker in Germany found that if the very energetic alpha particles emitted from polonium fell on certain light elements, specifically beryllium, boron, or lithium, an unusually penetrating radiation was produced. At first this radiation was thought to be gamma radiation, although it was more penetrating than any gamma rays known, and the details of experimental results were very difficult to interpret on this basis.[23][24] The next important contribution was reported in 1932 by Irène Joliot-Curie and Frédéric Joliot in Paris.[25] They showed that if this unknown radiation fell on paraffin, or any other hydrogen-containing compound, it ejected protons of very high energy. This was not in itself inconsistent with the assumed gamma ray nature of the new radiation, but detailed quantitative analysis of the data became increasingly difficult to reconcile with such a hypothesis.
In 1932, James Chadwick performed a series of experiments at the University of Cambridge, showing that the gamma ray hypothesis was untenable.[26] He suggested that the new radiation consisted of uncharged particles of approximately the mass of the proton, and he performed a series of experiments verifying his suggestion.[27] These uncharged particles were called neutrons, apparently from the Latin root for neutral and the Greek ending -on (by imitation of electron and proton).[28]
Positrons
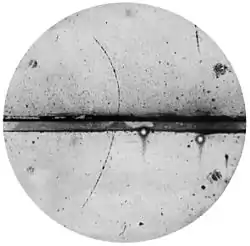
Dmitri Skobeltsyn first observed the positron in 1929.[29][30] While using a Wilson cloud chamber[31] to try to detect gamma radiation in cosmic rays, Skobeltsyn detected particles that acted like electrons but curved in the opposite direction in an applied magnetic field.[30]
Likewise, in 1929 Chung-Yao Chao, a graduate student at Caltech, noticed some anomalous results that indicated particles behaving like electrons, but with a positive charge, though the results were inconclusive and the phenomenon was not pursued.[32]
Carl D. Anderson discovered the positron on August 2, 1932,[33] for which he won the Nobel Prize for Physics in 1936.[34] Anderson also coined the term positron. The positron was the first evidence of antimatter and was discovered when Anderson allowed cosmic rays to pass through a cloud chamber and a lead plate. A magnet surrounded this apparatus, causing particles to bend in different directions based on their electric charge. The ion trail left by each positron appeared on the photographic plate with a curvature matching the mass-to-charge ratio of an electron, but in a direction that showed its charge was positive.
Neutrinos
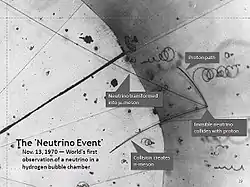
The neutrino more specifically, the electron neutrino was postulated first by Wolfgang Pauli in 1930 to explain how beta decay could conserve energy, momentum, and angular momentum (spin).
Enrico Fermi coined "neutrino" in 1933 to distinguish between the neutron and the much lighter neutrino.[35][Niels Bohr was notably opposed to this interpretation of beta decay and was ready to accept that energy, momentum and angular momentum were not conserved quantities.]
- n0
→ p+
+ e−
+ ν
e
In 1942 Wang Ganchang first proposed the use of beta-capture to experimentally detect neutrinos.[36] In the July 20, 1956 issue of Science, Clyde Cowan, Frederick Reines, F. B. Harrison, H. W. Kruse, and A. D. McGuire published confirmation that they had detected the neutrino,[37][38] a result that was rewarded almost forty years later with the 1995 Nobel Prize.[39]
"In this experiment, now known as the Cowan–Reines neutrino experiment, antineutrinos created in a nuclear reactor by beta decay reacted with protons producing neutrons and positrons:
- ν
e + p+
→ n0
+ e+
The positron quickly finds an electron, and they annihilate each other. The two resulting gamma rays (γ) are detectable. The neutron can be detected by its capture on an appropriate nucleus, releasing a gamma ray. The coincidence of both events – positron annihilation and neutron capture – gives a unique signature of an antineutrino interaction.
Electromagnetics
"Direct observation of [Dark Matter] DM halos is impossible with conventional (read electromagnetic radiation) astronomy, and so a common method for investigating DM halos is use of N-body computer simulations of the formation of galaxy-sized systems of DM particles."[40]
Gamma rays
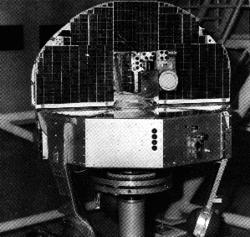
Long before experiments could detect gamma rays emitted by cosmic sources, scientists had known that the universe should be producing these photons. Work by Eugene Feenberg and Henry Primakoff in 1948, Sachio Hayakawa and I.B. Hutchinson in 1952, and, especially, Philip Morrison in 1958[41] had led scientists to believe that a number of different processes which were occurring in the universe would result in gamma-ray emission. These processes included cosmic ray interactions with interstellar gas, supernova explosions, and interactions of energetic electrons with magnetic fields. However, it was not until the 1960s that our ability to actually detect these emissions came to pass.[42]
The first gamma-ray telescope carried into orbit, on the Explorer 11 satellite in 1961, picked up fewer than 100 cosmic gamma-ray photons. They appeared to come from all directions in the Universe, implying some sort of uniform "gamma-ray background". Such a background would be expected from the interaction of cosmic rays (very energetic charged particles in space) with interstellar gas.
"[T]he University of Minnesota Gamma-ray Experiment [aboard OSO 1] was designed to provide preliminary measurements of the intensity and directional properties of low-energy gamma-rays in space. The detector operated in the 50 keV - 3 MeV range. For the 50-150 keV range, a NaI(Tl) scintillation crystal monitored radiation through a lead shield. The detector operating in the 0.3-1.0 MeV and 1.0-3.0 MeV energy regions used two scintillators connected as a Compton coincidence telescope. ... The U. Minnesota gamma-ray experiment on OSO 1 produced a measurement of the extraterrestrial gamma-ray flux between 0.5-3.0 MeV, and an indication of its origin on the celestial sphere. Equally important, this experiment began to define the background problems encountered in gamma-ray astronomy."[43] OSO 1 is launched on March 7, 1962.[43]
The first true astrophysical gamma-ray sources were solar flares, which revealed the strong 2.223 MeV line predicted by Morrison. This line results from the formation of deuterium via the union of a neutron and proton; in a solar flare the neutrons appear as secondaries from interactions of high-energy ions accelerated in the flare process. These first gamma-ray line observations were from OSO-3 and OSO-7.
"Gamma radiation astronomy only developed after specialized satellites had been put into outer space."[44]
"France and the U.S.S.R. are cooperating in a gamma-radiation astronomy project called Sigma (gamma imaging system resolution with aleatory masking). The mission of Sigma is to map the heavens in the soft gamma range and in the hard X-ray range."[45]
"Not until 1968 do gamma rays blossom in ApJ, the traditional bastion of conservative astronomy. Neutrino and gravitational radiation astronomy have had comparable checkered beginnings in roughly the same time period. Astronomers still seem to prefer the photon they know to the photon they don't."[46]
X-rays
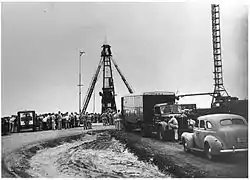
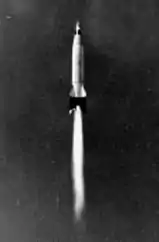
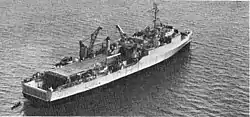
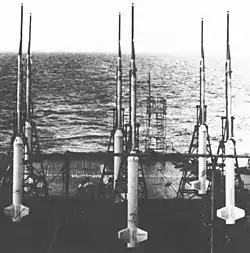
In 1927, E.O. Hulburt of the US Naval Research Laboratory (NRL) and associates Gregory Breit and Merle Tuve of the Carnegie Institution of Washington considered the possibility of equipping Robert H. Goddard's rockets to explore the upper atmosphere.[47] "Two years later, he proposed an experimental program in which a rocket might be instrumented to explore the upper atmosphere, including detection of ultraviolet radiation and X-rays at high altitudes."[47]
In the late 1930s, "the presence of a very hot, tenuous gas surrounding the Sun ... was inferred indirectly from optical coronal lines of highly ionized species".[48] In the mid-1940s "radio observations revealed a radio corona" around the Sun.[48] "Of course, the sheer beauty of the solar corona has been admired in scattered visible light ever since humans first wondered about solar eclipses"[48].
The ending of World War II presented an opportunity to use a ballistic missile for just such a purpose. The White Sands Proving Grounds in New Mexico, at the time an army base, is the first location on land to test the concept. The image at the right shows the V-2 launch complex prior to the launch of V-2 number 6.
The beginning of the search for X-ray sources above the Earth's atmosphere is August 5, 1948, at 12:07 GMT (Greenwich Mean Time).[49][50] As part of Project Hermes a US Army (formerly German) V-2 rocket number 43 is launched from White Sands Proving Grounds, launch complex (LC) 33, to an altitude of 166 km.[50] This is "the first detection of solar X-rays."[51]
The USS Point Defiance (LSD-31) is one of the first rocket-launching surface ships to support the 1958 IGY Solar Eclipse Expedition to the Danger Island region of the South Pacific. Launchers on deck fired eight Nike-Asp sounding rockets. Each rocket carried an X-ray detector to record X-ray emission from the Sun during the solar eclipse on October 12, 1958.
Ultraviolets
In 1801, the German physicist Johann Wilhelm Ritter made the discovery of ultraviolet by noting that the rays from a prism darkened silver chloride preparations more quickly than violet light. Ritter's experiments were an early precursor to what would become photography. Ritter noted that the UV rays were capable of causing chemical reactions.
The discovery of the ultraviolet radiation below 200 nm, named vacuum ultraviolet because it is strongly absorbed by air, was made in 1893 by the German physicist Victor Schumann.[52]
Opticals

Based only on uncertain descriptions of the first practical telescope, invented by Hans Lippershey in the Netherlands in 1608, Galileo, in the following year, made a telescope with about 3x magnification. He later made improved versions with up to about 30x magnification.[53] With a Galilean telescope the observer could see magnified, upright images on the earth—it was what is commonly known as a terrestrial telescope or a spyglass. He could also use it to observe the sky; for a time he was one of those who could construct telescopes good enough for that purpose. On 25 August 1609, he demonstrated one of his early telescopes, with a magnification of about 8 or 9, to Venetian lawmakers. His telescopes were also a profitable sideline for Galileo selling them to merchants who found them useful both at sea and as items of trade. He published his initial telescopic astronomical observations in March 1610 in a brief treatise entitled Sidereus Nuncius (Starry Messenger).[54]
Visuals
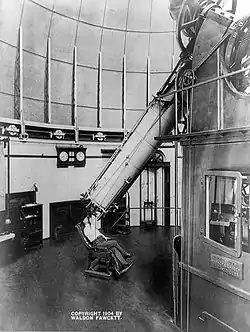
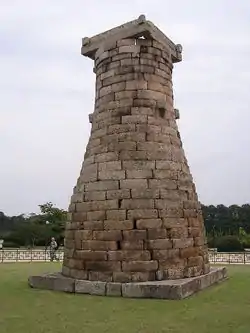
What is “the “old-fashioned” spirit of real-time visual astronomy”?[55] “I think everyone can conjure up a mental image of astronomers at every level and place in history, gazing through the eyepieces of their telescopes at sights far away - true visual astronomy.”[55]
In popular culture optical astronomy encompasses a wide variety of observations via telescopes that are sensitive in the range of visible light. Scientists would call this visible-light astronomy. It includes imaging, where a picture of some sort is made of the object; photometry, where the amount of light coming from an object is measured, spectroscopy, where the distribution of that light with respect to its wavelength is measured, and polarimetry where the polarisatio] state of that light is measured.
Pretelescopic astronomy is the science of observing celestial objects with the naked eye.
Some of the pretelesopic astronomers were the Chinese due to evidence such as the Gan Shi Xing Jing (the oldest recorded star catalog which was produced during the 5th century BCE).
The five oldest, extant observatories according to NASA are as follows:
- Abu Simbel, Egypt
- Stonehenge, Great Britain
- Angkor Wat, Cambodia
- Kokino, Republic of Macedonia
- Goseck circle, Germany
Violets

Violet photographs of the planet Venus taken in 1927 “recorded two nebulous bright streaks, or bands, running ... approximately at right angles to the terminator” that may be from the upper atmosphere.[56]
In 1959 "observations of the spectrum of the planet Venus, with spectrographs of low and high dispersion at the Georgetown College Observatory, show that a wide, continuous absorption band is present in the violet and near-ultraviolet."[57]
The image at right is from the Galileo spacecraft solid state imaging system taken on February 14, 1990. The satellite was about 2.7 million km from the planet. The highpass violet filter (418 nm) has been applied to emphasize the smaller scale cloud features. This rendition has been colorized bluish to emphasize subtle contrasts in the cloud markings. The sulfuric acid clouds indicate considerable convective activity. The filamentary dark features are composed of several dark nodules, like beads on a string, each about 96 km across.
Cyans
One of the substances discovered in the tail by spectroscopic analysis was the toxic gas cyanogen,[58] which led astronomer Camille Flammarion to claim that, when Earth passed through the tail, the gas "would impregnate the atmosphere and possibly snuff out all life on the planet."[59]
On July 1, 1957, "Following the intense auroral display of the previous night, ... The variation in Hβ emission ... shows quite clearly that the sudden transition from an [auroral] arc to rays coincides with a decrease in the intensity of the hydrogen emission and an inversion of the polarity of the magnetic disturbance."[60]
Yellows
Io and Europa were seen for the first time as separate bodies during Galileo's observations of the Jupiter system the following day, January 8, 1610 (used as the discovery date for Io by the [International Astronomical Union] IAU).[61] The discovery of Io and the other Galilean satellites of Jupiter was published in Galileo's Sidereus Nuncius in March 1610.[62]
Oranges
According to the renowned double star observer Robert Aitken (1961), Father Richaud discovered Alpha Centauri AB's duplicity from the Indian city of Pondicherry in December 1689 while observing a comet.[63][64] By 1752, French astronomer Abbé Nicolas Louis de Lacaille made astrometric positional measurements using a meridian circle while John Herschel, in 1834, made the first micrometrical observations.[65]
Alpha Centauri B is one of the earlier K spectral type stars observed.
Astronomers categorize Epsilon Eridani as a main-sequence star of spectral class K2, which means that energy is emitted from the surface at a temperature of about 5,000 K, giving the star an orange hue.
Epsilon Eridani, the Bayer designation for this star, was established in 1603 as part of the Uranometria, a star catalogue produced by German celestial cartographer Johann Bayer.
Reds
The Balmer series is named after Johann Balmer, who discovered the Balmer formula, an empirical equation to predict the Balmer series, in 1885.
“In 1926 ... [t]here were no national observatories (except the Naval Observatory), very little chance for guest observing elsewhere, no radio astronomy, no X-ray astronomy, no satellite astronomy, and very little infrared or even red astronomy!”[66] Bold added.
Infrareds
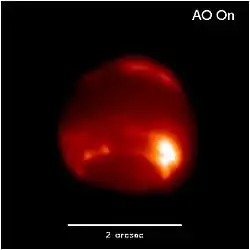
Infrared astronomy began in the 1830s, a few decades after the discovery of infrared light by William Herschel in 1800.
Herschel performed an experiment where he placed a thermometer in sunlight of different colors after it passed through a prism. He noticed that the temperature increase induced by sunlight was highest outside the visible spectrum, just beyond the red color. That the temperature increase was highest at infrared wavelengths was due to the spectral index of the prism rather than properties of the Sun, but the fact that there was any temperature increase at all prompted Herschel to deduce that there was invisible radiation from the Sun. He dubbed this radiation "calorific rays", and went on to show that it could be reflected, transmitted, and absorbed just like visible light.[67]
Efforts were made starting in the 1830s and continuing through the 19th century to detect infrared radiation from other astronomical sources. Radiation from the Moon was first detected in 1873 by William Parsons, 3rd Earl of Rosse. Ernest Fox Nichols used a modified Crookes radiometer in an attempt to detect infrared radiation from Arcturus and Vega, but Nichols deemed the results inconclusive. Even so, the ratio of flux he reported for the two stars is consistent with the modern value, so George Rieke gives Nichols credit for the first detection of a star other than our own in the infrared.[68]
Radars
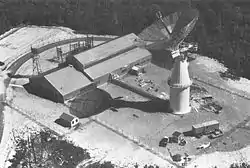
"The moon is comparatively close and was detected by radar, soon after the invention of the technique, in 1946.[69][70] Measurements included surface roughness and later mapping of shadowed regions near the poles.
Radios
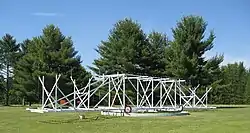
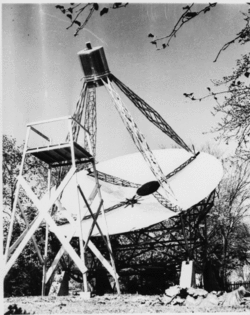
Radio waves were not detected first from a natural source, but were rather produced deliberately [and] artificially by the German scientist Heinrich Hertz in 1887, using electrical circuits calculated to produce oscillations in the radio frequency range, following recipies suggested by the equations of James Clerk Maxwell.
The first radio antenna used to identify an astronomical radio source was one built by Karl Guthe Jansky, an engineer with Bell Telephone Laboratories, in 1931.
The initial detection of radio waves from an astronomical object was made in the 1930s, when Karl Jansky observed radiation coming from the Milky Way.
An amateur radio operator, Grote Reber, was one of the pioneers of what became known as radio astronomy when he built the first parabolic "dish" radio telescope (9 m (30 ft) in diameter) in his back yard in Illinois in 1937. He was instrumental in repeating Karl Guthe Jansky's pioneering but somewhat simple work at higher frequencies, and he went on to conduct the first sky survey at very high radio frequencies (VHF).
Gravitationals
"Gravitational radiation astronomy should be the next contributor to this revolution in astrophysics and cosmology. The attempt to detect gravitational radiation is a difficult and exciting area of current research. LIGO has been built and is operating [1, 2]. Around the globe a worldwide network of detectors is coming online; TAMA in Japan [3] and GEO in Germany [4] are operating alongside LIGO in the quest for gravitational radiation detection, while VIRGO in Italy [5, 6] will soon also be online. The existence of gravitational radiation was predicted by Albert Einstein [7], and confirmed through the binary neutron star observations of Taylor and Weisberg [8]. The direct detection of gravitational radiation will be a tremendous accomplishment for physics, but it will almost certainly also cause an upheaval in our knowledge of the universe and how it works. The observations of expected sources should be dramatic: supernovae, pulsars, the inspiral of binary neutron stars followed by black-hole formation, or even the stochastic background from the Big Bang."[71]
"An epoch-making solid laser is developed. It is ceramic laser, polycrystal, which is produced as same as glass and shows ultra high output. Ti3+:Al2O3 laser crystal and the CPA (chirped pulse amplification) technique realized new ultra high output lasers. Japan has developed various kinds of ceramic lasers, from 10-2 to 67 x 103 w average output, since 1995. These ceramic lasers were studied by gravitational radiation astronomy."[72]
"Some of the experimental scientists may have felt they were testing a global theory some of the time, but sometimes they were also “getting a foot-hold” in the early stages of gravitational radiation astronomy, developing their instrumentation (detectors were built at Hughes Aircraft, IBM and Bell Labs), keeping up with the physics, trying to kill off an irritating nuisance who was making too much noise about some unbelievable results (see [Collins 1981a]), confirming the existence of gravity waves in the face of mindless criticism and much more besides."[73]
"Now, gravity waves are emitted strongly in regions of great space-time curvature, regions associated [with] compact objects such as white dwarfs, neutron [stars], black holes supernovae cores about which information is difficult to obtain [by] established electromagnetic radiation astronomy (eg X-ray, [...] ultraviolet, optical, and gamma ray astronomy."[74]
Sun
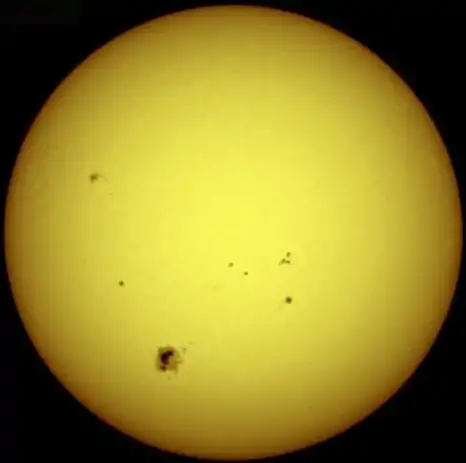
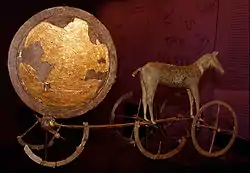
5102 b2k, -3102 or 3102 BC, is the historical year assigned to a Hindu table of planets that does include the classical planet, the Sun.[75]
~3000 b2k: In the early first millennium BCE, Babylonian astronomers observed that the Sun's motion along the ecliptic was not uniform.
~2300 b2k: Eratosthenes estimated the distance between the Earth and the Sun in the 3rd century BCE as "of stadia myriads 400 and 80000", the translation of which is ambiguous, implying either 4,080,000 Stadiametric rangefinding stadia (755,000 km) or 804,000,000 stadia (148 to 153 million kilometers or 0.99 to 1.02 AU); the latter value is correct to within a few percent. The theory that the Sun is the center around which the planets move was first proposed by the ancient Greek Aristarchus of Samos in the 3rd century BCE.
Worship of the Sun was central to civilizations such as the Inca of South America and the Aztecs of what is now Mexico. Many ancient monuments were constructed with solar phenomena in mind; for example, stone megaliths accurately mark the summer or winter solstice (some of the most prominent megaliths are located in Nabta Playa, Egypt; Mnajdra, Malta and at Stonehenge, England); Newgrange, a prehistoric human-built mount in Ireland, was designed to detect the winter solstice; the pyramid of El Castillo at Chichén Itzá in Mexico is designed to cast shadows in the shape of serpents climbing the pyramid at the vernal and autumn equinoxes.
Coronal clouds

"[T]he solar corona is eminently variable, and therefore like our aurora borealis, which is known to be electric."[76] "This vast electric mass must have a great electric repulsion through vacant space, and it lends probability to my position that it drives away from the sun the tails of comets and our zodiacal light and aurora borealis."[76] "Electricity alone can repel electricity."[76] "[T]he direction of the comets' tails is but the interaction between the sun and the comets, the same as the action between a charged prime conductor and a charged pith ball of an electric machine."[76]
"[A] variety of geophysical and astrophysical phenomena can be explained by a net charge on the Sun of -1.5 x 1028 e.s.u."[77] This figure was later reduced by a factor of five.[78]
"There appears to be considerable misunderstanding on the part of physicists of the nature and degree of the observational support of gravitational theory. For example, it appears to be commonly believed that the observations of planetary motion agree with computed orbits to the accuracy of the observations. On the other hand, it has long been known by the astronomers that there are sizable systematical discrepancies between computed and observed orbits".[79]
"Beginning with the daguerreotype of the corona of 1851, the Reverend Lecturer had thrown on the screen pictures illustrating the form of the corona in different years. The drawings of those of 1867, 1878, and 1900 all showed long equatorial extensions with openings at the solar poles filled with beautiful rays."[80] "The intermediate years, as, for example, 1883, 1886, and 1896 showed the four groups of synclinals which mainly constitute the corona gradually descending towards the equator of the sun, with a corresponding opening of the polar regions."[80]
"Some of the theories of the solar corona were then illustrated and discussed."[80]
- "The corona is not of the nature of an atmosphere round the sun, for the pressure at the sun's limb would be enormous, while the thinness of the chromospheric lines show that it is not."[80]
- "comets, such as that of 1843, have approached the sun with enormous velocities within the region of the prominences without suffering disruption or retardation."[80]
- "If not an atmosphere of particles of gas, still less is it an atmosphere of solid stones or meteorites."[80]
- "Meteor streams do circle round the sun, but there is no reason why the positions of the orbits, or the intrinsic brightness of such streams should vary with the sun-spot period."[80]
- "the appearance of the corona does not seem to be such as the projection of meteor streams upon the celestial vault would give."[80]
- "Prof. Schaeberle has proposed a mechanical origin of the solar corona, due to the forces of ejection of particles from the solar limb, as evidenced by the prominences, and the force of gravity under the particular conditions of the solar rotation and the inclination of its axis to the earth's orbit."[80]
- "The electrical theory of the corona does not negative the mechanical theory, but supplements it. In addition to the forces of gravity and ejection, it takes account of the repulsive force which the sun exerts on matter which has the same electrical sign as itself, and which has been ejected from it."[80]
- "it would seem that the solar corona is of the nature of an electrical aurora round the sun."[80]
- "the coronoidal discharges in poor vacua obtained by Prof. Pupin about an insulated metal ball are exceedingly like the rays and streamers of the solar corona."[80]
Initially the concept of a coronal cloud developed with observations of clouds or cloud-like structures forming above the photospheric surface of the Sun.
"Coronal clouds, type IIIg, form in space above a spot area and rain streamers upon it."[81] Type IIIg is a subdivision of sunspot prominences (class III).[81] "Occasionally dots form in space above a sunspot group. Other dots then appear, and all coalesce into a suspended cloud from which streamers pour into the spot area."[81]
"Occasionally one member of an interactive group has been a tornado prominence, but, for the first time, on January 4, 1945, a coronal cloud prominence of the sunspot class was observed to participate in such an interaction."[82] "The coronal cloud prominence ... formed in space, and from it streamers flowed into a neighboring prominence instead of into a spot area on the sun as is usual with coronal prominences."[82] "On January 5, a surge was photographed over the spot area, the coronal cloud being still visible. On January 6, a trace of the cloud was visible, but solar rotation soon carried the whole interactive group from the limb. The coronal cloud probably endured at least 2 days."[82]
"On December 18, 1956, a large and active sunspot region was at the west limb of the sun."[83] "Continuous emission from a dense coronal cloud was observed just before the flare. This cloud condenses sharply during the flare and dissipates afterward."[83] After a second class 2 flare occurred at 2131 U.T., "[a]t about 2200 U.T. a bright cloud appeared above the limb at the active region, reaching maximum surface brightness at 2208 and maximum total brightness at around 2220."[83] "The flare at 2200 (the bright cloud) appears larger and brighter on the coronagraph photographs, but we are definitely looking at the same object."[83] The initial "flare appears to have been a violent condensation of material from a dense coronal cloud above an active region."[83] "The coronal temperature was 4000000°."[83]
Solar cycles
"For the greater part of the sun-spot period there is practically but one zone of spots in each hemisphere. The departure from this condition of things near or at the time of minimum, when the spots of the dying cycle are approaching the equator, and the forerunners of the new cycle are beginning to appear in high latitudes, is the only case in which the solar spots are distinctly separated into more than a single zone in each hemisphere."[84] "Spöerer's law ... involves that in a minimum year the zone about 15° should be entirely barren".[84]
"First of all there were only fifteen groups seen during the entire year [1901], north and south put together. Of these, seven were in the north, and the mean latitude for the north was 8.6°, exactly the latitude of one spot of the seven, and this very naturally, seeing that it was by far the greatest group of the year, the celebrated "eclipse group.""[84] Bold added. "Greatest group" and "eclipse group" are both relative synonyms for "dominant group".
"[T]he spot-groups have been carefully examined for cases of return, and where it appeared clear that the same group has returned a second time or more frequently, without any temporary disappearance or subsidence, such a long-continued group has been treated as an entity throughout."[85] Bold added. "It has been forgotten that, whatever the cause which produces this variation of rotation rate with latitude, the causes producing difference of rate within any given latitude are more effective still."[85]
"[T]here is a slight retardation of the rotation period from the first cycle to the second, shown by both northern and southern hemispheres."[85]
Mercury

5102 b2k, is the historical year assigned to a Hindu table of planets that does include the classical planet Mercury (or Hermes).[75] "Hermès observait en -1660; donc les Indiens observaient en -3102, et ils observaient bien!"[75]
~ 3300 b2k: The earliest known recorded observations of Mercury are from the Mul.Apin tablets. These observations were most likely made by an Assyrian astronomer around the 14th century BC.[86] The cuneiform name used to designate Mercury on the Mul.Apin tablets is transcribed as Udu.Idim.Gu\u4.Ud ("the jumping planet").[87]
~2900 b2k: Babylonian records of Mercury date back to the 1st millennium BC. The Babylonians called the planet Nabu after the messenger to the gods in their mythology.[88]
Venus
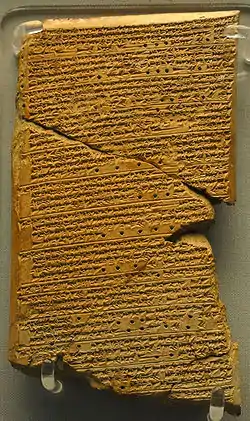
5102 b2k, -3102 or 3102 BC, is the historical year assigned to a Hindu table of planets that does not include the classical planet Venus.[75] "Vénus seule ne s'y trouvait pas."[75] "Venus alone is not found there."[89]
3581 b2k: The Venus tablet of Ammisaduqa, dated 1581 BC, shows that the Babylonians understood that the two were a single object, referred to in the tablet as the "bright queen of the sky," and could support this view with detailed observations.[90]
Zodiacal lights
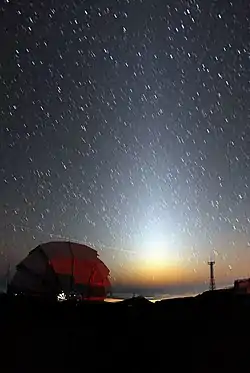
"According to Gruson and Brugsch the Egyptians were acquainted with, and even worshipped, the zodiacal light from the very earliest times, as a phenomenon visible throughout the East before sunrise and after sunset. It was described as a glowing sheaf or luminous pyramid perpendicular to the horizon in summer, and inclined more or less during the winter. Indeed the Egyptians represented the zodiacal light under the form of a triangle which sometimes stood upright and at other times was inclined."[91]
Moon
.gif)
Tally sticks, notched bones dating as far back as 20–30,000 years ago, are believed by some to mark the phases of the Moon.[92][93][94]
5102 b2k, is the historical year assigned to a Hindu table of planets that does include the classical planet, the Moon.[75]
~2500 b2k: “Understanding of the Moon's cycles was an early development of astronomy: by the 5th century BC, Babylonian astronomers had recorded the 18-year Saros cycle of lunar eclipses,[95] The texts discussed in that article are more recent than 490 BC and, as mentioned in the paper, the observations can have occurred no earlier than that. The earliest reference for the Metonic cycle in Neugubauer's (1957) The Exact Sciences in Antiquity is 380 BC (p. 140).
Indian astronomers had described the Moon’s monthly elongation.[96]
The Chinese astronomer Shi Shen (fl. 4th century BC) gave instructions for predicting solar and lunar eclipses.[97] ... Although the Chinese of the Han Dynasty believed the Moon to be energy equated to qi, their 'radiating influence' theory also recognized that the light of the Moon was merely a reflection of the Sun, and Jing Fang (78–37 BC) noted the sphericity of the Moon.[97]
"The categories of radiation activity are charged particle investigations, magnetic field investigations, and radiation-astronomy experiments."[98]
Mars
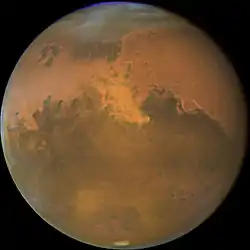
5102 b2k is the historical year assigned to a Hindu table of planets that does not include the classical planet Venus.[75] "Vénus seule ne s'y trouvait pas."[75] Mars is mentioned.[75]
~3534 b2k The existence of Mars as a wandering object in the night sky was recorded by the ancient Egyptian astronomers and by 1534 BCE they were familiar with the retrograde motion of the planet.[99]
~3458 b2k: Mars was portrayed on the ceiling of the tomb of Seti I and on the Ramesseum ceiling, though it was missing from the Senenmut star map Hatshepsut reigned (1479 BC – 1458 BC). In the latter case, the planet may have been in conjunction with the Sun.[100]
3045 b2k: Chinese records about the appearances and motions of Mars appear from before the founding of the Zhou Dynasty (1045 BCE).
ca. 2626 b2k: By the period of the Neo-Babylonian Empire, the Babylonian astronomers were making regular records of the positions of the planets and systematic observations of their behavior. For Mars, they knew that the planet made 37 synodic periods, or 42 circuits of the zodiac, every 79 years. They also invented arithmetic methods for making minor corrections to the predicted positions of the planets.[101][102]
Jupiter
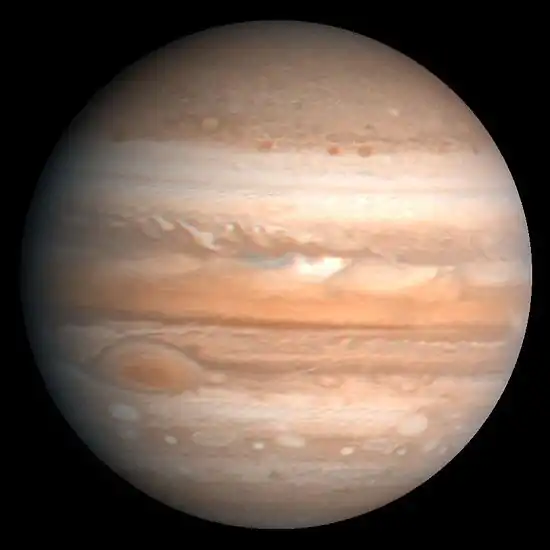
5102 b2k, -3102 or 3102 BC, is the historical year assigned to a Hindu table of planets that does include the classical planet Jupiter.[75]
~2800 b2k: The observation of Jupiter dates back to the Babylonian astronomers of the 7th or 8th century BC.[103] To the Babylonians, this object represented their god Marduk. They used the roughly 12-year orbit of this planet along the ecliptic to define the constellations of their zodiac.[104][105]
2362 b2k: The Chinese historian of astronomy, Xi Zezong, has claimed that Gan De, a Chinese astronomer, made the discovery of one of Jupiter's moons in 362 BC with the unaided eye. The Chinese, Korean and Japanese referred to the planet as the wood star.
Saturn
.jpg.webp)
“Saturn has been known since prehistoric times.[106]
Apparently 5102 b2k (before the year [Epoch astronomy] 2000.0), -3102 or 3102 BC, is the historical year assigned to a Hindu table of planets that does include the classical planet Saturn.[75]
Babylonian astronomers systematically observed and recorded the movements of Saturn.[107]
Ancient Chinese and Japanese culture designated the planet Saturn as the earth star.
Solar binary
"Since the invention of the telescope, many pairs of double stars have been found. Early examples include Mizar and [Alpha Crucis] Acrux. Mizar, in the Big Dipper (Ursa Major), was observed to be double by Giovanni Battista Riccioli in 1650[108][109] (and probably earlier by Benedetto Castelli and Galileo).[110] The bright southern star Acrux, in the Southern Cross, was discovered to be double by Father Fontenay in 1685.[108]"
Comets
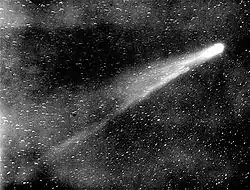
"The 1910 approach, which came into naked-eye view around 10 April[111] and came to perihelion on 20 April,[111] was notable for several reasons: it was the first approach of which photographs exist, and the first for which spectroscopic data were obtained.[112] Furthermore, the comet made a relatively close approach of 0.15AU,[111] making it a spectacular sight. Indeed, on 19 May, the Earth actually passed through the tail of the comet.[113][114] One of the substances discovered in the tail by spectroscopic analysis was the toxic gas cyanogen,[58] which led astronomer Camille Flammarion to claim that, when Earth passed through the tail, the gas "would impregnate the atmosphere and possibly snuff out all life on the planet."[59] His pronouncement led to panicked buying of gas masks and quack "anti-comet pills" and "anti-comet umbrellas" by the public.[115] In reality, as other astronomers were quick to point out, the gas is so diffuse that the world suffered no ill effects from the passage through the tail.[59]
"It is quite possible that [faint streamers preceding the main tail and lying nearly in the prolonged radius vector] may have touched the Earth, probably between May 19.0 and May 19.5, [1910,] but the Earth must have passed considerably to the south of the main portion of the tail [of Halley's comet]."[116]
Milky Way
Democritus "lived at Abdère 300 years before the Christian era [2300 b2k]. In a short fragment quoted by Plutarch, he declares that the Milky Way is an agglomeration of small stars too far away to be perceived singly."[117]
Andromeda galaxy
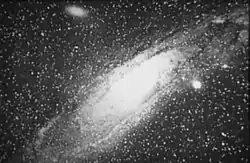
Beyond the confines of our galaxy is the Andromeda galaxy.
The earliest recorded observation of the Andromeda Galaxy was in 964 CE by the Persian astronomer, Abd al-Rahman al-Sufi (Azophi),[118] who described it as a "small cloud" in his Book of Fixed Stars. Other star charts of that period have it labeled as the Little Cloud.[118]
History
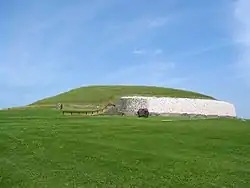
Today, astronomical history is usually divided into three parts: archaeoastronomy, historical astronomy, and the history of astronomy. These divisions are sincerely based on the belief that the trained astronomers of today are different from the observers of antiquity.
Archaeoastronomy (also spelled archeoastronomy) is the study of how people in the past "have understood the phenomena in the sky how they used phenomena in the sky and what role the sky played in their cultures."[119] Clive Ruggles argues it is misleading to consider archaeoastronomy to be the study of ancient astronomy, as modern astronomy is a scientific discipline, while archaeoastronomy considers other cultures' symbolically rich cultural interpretations of phenomena in the sky.[120][121]
Historical astronomy
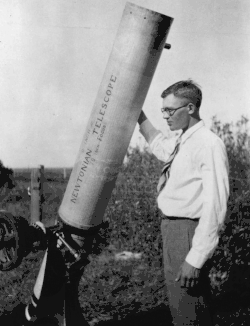
Historical astronomy is the science of analysing historic astronomical data. The American Astronomical Society (AAS), established [in] 1899, states that its Historical Astronomy Division "...shall exist for the purpose of advancing interest in topics relating to the historical nature of astronomy. By historical astronomy we include the history of astronomy; what has come to be known as archaeoastronomy; and the application of historical records to modern astrophysical problems."[122] Historical and ancient observations are used to track theoretically long term trends, such as eclipse patterns and the velocity of nebular clouds.[123] Conversely, utilizing known and well documented phenomenological activity, historical astronomers apply computer models to verify the validity of ancient observations, as well as Astronomical chronology dating such observations and documents which would otherwise be unknown.
Prehistory
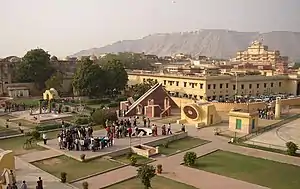
The prehistory period dates from around 7 x 106 b2k to about 7,000 b2k.
Astronomy is the oldest of the natural sciences, dating back to antiquity, with its origins in the religious, mythological, and astrological practices of pre-history: vestiges of these are still found in astrology, a discipline long interwoven with public and governmental astronomy, and not completely disentangled from it until a few centuries ago in the Western World (see astrology and astronomy). In some cultures astronomical data was used for astrological prognostication. Ancient astronomers were able to differentiate between stars and planets, as stars remain relatively fixed over the centuries while planets will move an appreciable amount during a comparatively short time.
Ancient history
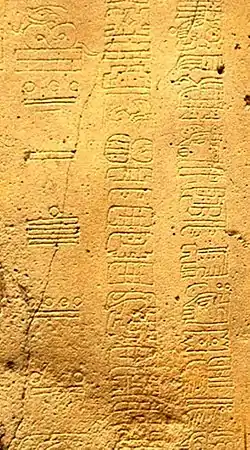
The ancient history period dates from around 8,000 to 3,000 b2k.
"Sumerian astronomers were the first to keep written records of what they learned about the heavens."[124] A form of writing known as cuneiform emerged among the Sumerians around 3500–3000 BC, 5500-5000 b2k.
The modern scientific discipline of astronomy focuses on reproducibility and physical theory to explain and describe observations. Many ancient observers produced remarkably reproducible calendars such as the Mesoamerican Long Count calendar which apparently goes from August 11, 3114 [Common era] BCE, to October 13, 4772, and beyond.
Historians consider "that "[the Chinese] were the most persistent and accurate observers of celestial phenomena anywhere in the world before the Arabs."[125] Star names later categorized in the twenty-eight mansions have been found on oracle bones unearthed at Anyang, dating back to the middle Shang Dynasty (Chinese Bronze Age), and the mansion (xiù:) system's nucleus seems to have taken shape by the time of the ruler Wu Ding (1339-1281 BC, [3339-3281 b2k]).[126] It is believed that the first pretelescopic astronomers were the Chinese due to conclusive evidence such as the Gan Shi Xing Jing (the oldest recorded star catalog which was produced during the 5th century BCE).
Early history
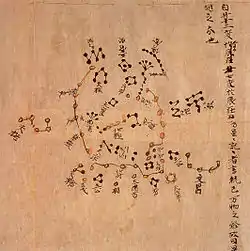
"About 280 B.C. [2280 b2k], ... Aristarchus of Samos proposed the hypothesis that the Sun is at rest, while the Earth and the planets rotate about the Sun."[127] "Aristarchus also figured out how to measure the distances to the Sun and the Moon and their sizes."[128]
The celestial sphere may have been produced very early: According to records, the first celestial globe was made by Geng Shou-chang () between 70 BC and 50 BC. In the Ming Dynasty, the celestial globe at that time was a huge globe, showing the 28 mansions, celestial equator and ecliptic. None of them have survived.
The Dunhuang map from the Tang Dynasty of the North Polar region at right is thought to date from the reign of Emperor Zhongzong of Tang (705–710). Constellations of the three schools are distinguished with different colors: white, black and yellow for stars of Wu Xian, Gan De and Shi Shen respectively. The whole set of star maps contains 1,300 stars.
The divisions of history for astronomy are sincerely based on the belief that the trained astronomers of today are different from the observers of antiquity. One fundamental differentiator is technology.
A big boon to visual astronomy is the telescope. The first known practical telescopes were invented in the Netherlands at the beginning of the 1600s (the 17th century), using glass lenses. And, on this instrument of astronomy, there is this: "There are indeed ancient tablets that mention astronomers' lenses supported by a golden tube to enlarge the pupil, and in Nineveh a rock crystal lens was found (Pettinato 1998). Maybe one day a new archaeological excavation will find a Babylonian telescope for the first time."[129]
Computers
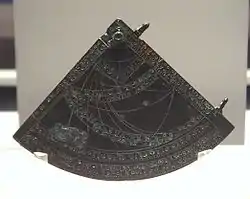
The astrolabe was effectively an analog calculator capable of working out several different kinds of problems in spherical astronomy.
Some form of an "astrolabe" may have been in use by the third millennium BC.[6]
Information science
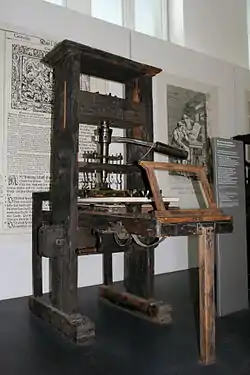
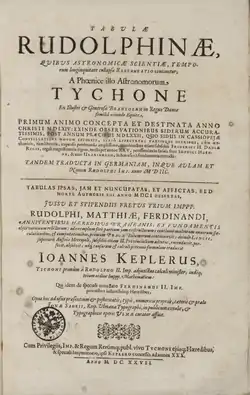
The invention of the printing press (an early example is shown at right) made it possible for scientists and politicians to communicate their ideas with ease, leading to the Age of Enlightenment; an example of technology as a cultural force.
At left is a copy of the title page of Johannes Kepler's Rudolphine Tables (1627). It is regarded as the most accurate and comprehensive star catalogue and planetary tables published up until that time. It contained the positions of over 1000 stars and directions for locating the planets within our solar system. Kepler finished the work in 1623 and dedicated it to his patron, the Emperor Rudolf II, but actually published it in 1627. The table's findings support Kepler's laws and the theory of a heliocentric astronomy.
Observation post
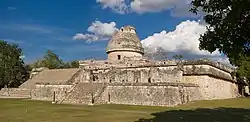
An observation post, temporary or fixed, is any preselected position from which observations are to be made - this may include very temporary installations or even an airborne aircraft.[130][131]
Historically, observatories were as simple as containing an astronomical sextant (for measuring the distance between stars) or Stonehenge (which has some alignments on astronomical phenomena).
Observatories
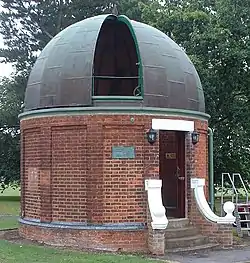
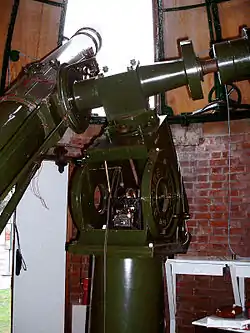
In 1891, Patrick Alexander ordered the telescope from the well-known Victorian telescope makers Thomas and Howard Grubb of Dublin with all the usual fittings associated with a professional instrument of the time.
In 1905 Patrick Alexander offered his telescope to the War Office, again including an offer to pay for the construction of an observatory. The offer was accepted and a site was chosen. Building work commenced with pegging out in 1906 with the bulk of the work being carried out by local labour. Work proceeded apace until a long, accurate straight edge was required so that the dome could be correctly positioned. Finding such an edge proved difficult and attracted some publicity at the time. The problem was soon overcome. On August 26, 1906 the dome, which also had been designed by Grubb, was installed and over the next three months, the telescope was put in place and final construction work was completed.
In December 1906 the Aldershot Observatory was officially opened.
“The location of the observatory can hardly be considered ideal for astronomical observations, even at the time of its construction. It is at a low elevation in an essentially urban setting of an army town with many nearby buildings that date from the time of its construction.[2] It is very near a road that is lit by streetlights, although this was somewhat ameliorated by a clockwork switch inside the observatory that would turn off the nearest streetlights for about 20 minutes. This clockwork system was upgraded in 1987. As the electricity supply has been removed in 2006, this facility is no longer available. ... In its current location, the observatory will be an island in a sea of houses and some people fear that it will be targeted by vandals or, perhaps, will have to be protected with high, unsightly fences.
Telescopes
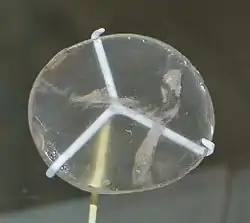
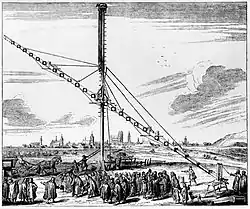
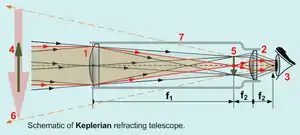
"Lens-shaped crystals have long been known from Bronze Age [5600-3300 b2k] contexts"[132]. These are "usually recognized as short-focus magnifying lenses."[132]
A range of sizes for planoconvex crystalline lenses has been discovered since about 1600 BC (3600 b2k),[132] where b2k is notation for 'before the year 2000'. Glass biconvex lenses have been discovered from 43-50 AD (1957-1950 b2k).[132]
The Keplerian Telescope, invented by Johannes Kepler in 1611, is an improvement on Galileo's design.[133] It uses a plano-convex lens as the eyepiece instead of Galileo's double concave one. The advantage of this arrangement is that the rays of light emerging from the eyepiece are converging. This allows for a much wider field of view and greater eye relief but the image for the viewer is inverted. Considerably higher magnifications can be reached with this design but to overcome aberrations the simple objective lens needs to have a very high focal ratio, or f-ratio.
"There are indeed ancient tablets that mention astronomers' lenses supported by a golden tube to enlarge the pupil, and in Nineveh a rock crystal lens was found (Pettinato 1998). Maybe one day a new archaeological excavation will find a Babylonian telescope for the first time."[129]
While finding an ancient tube telescope would be wonderful, air telescopes are a possibility.
Chronometers
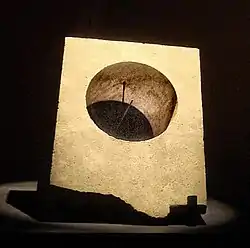
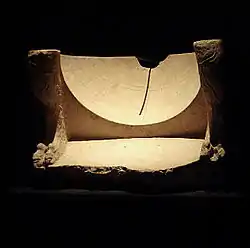
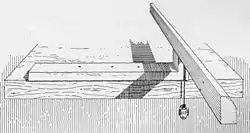
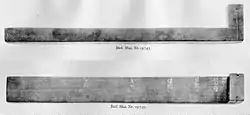
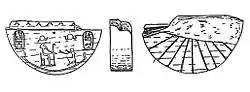
The image at right shows a sun dial from Ai Khanoum, Afghanistan, dated to the 3rd century BCE, ~2300 b2k. The image at left is also from Ai Khanoum, Afghanistan, showing its workings.
"[T]he earliest known sundial [is] from an Egyptian burial dated in the fifteenth century B.C. Sometimes called a shadow clock, or an L-board because of its shape [with] relatively crude performance."[134] A "[f]ragment of a late Egyptian sundial [from] about 3000 B.C." exists.[134]
At lower right is a diagram reconstructing the Egyptian Setjat for indicating hours of the morning or afternoon. No measurement was made until sunrise for a sufficient visual shadow to appear on the lower horizontal board. To indicate the first four hours, the head should be facing east and the board carefully leveled with plumb. After these four hours, the head is turned westward to measure four more hours. The two hours after sunset are not measured.
Lower left shows two views of an L-board. It is the oldest known sundial from the reign of Thutmose III (1479-1425 BC). The crossbar is missing. It has six imprints allowing measurement over ten hours. "Nicht messbar waren mit dieser Sonnenuhr die Zeiträume vor Sonnenaufgang und nach Sonnenuntergang, doch war dies hinsichtlich der Tageseinteilung auch nicht notwendig, da der altägyptische Tag mit Sonnenaufgang begann und mit Sonnenuntergang endete."
Lowest right is an Egyptian vertical sundial discovered at Gezer in Palestine. It is carved on a small piece of ivory 7 cm in diameter, engraved with the name of pharoh Merneptah. There are thirteen radial lines, each successive pair is at a constant angle of 15°, [t]reize lignes radiales, chaque paire successive formant un angle constant de 15°, étaient à l'origine gravées sur l'une des faces de l'horloge.
Lofting technology
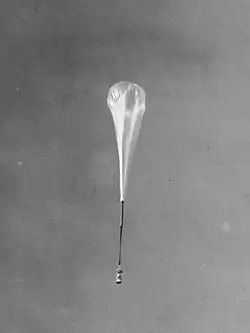
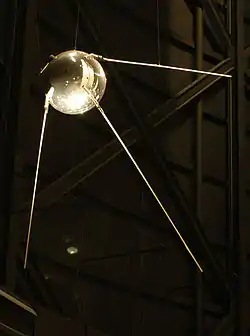
The Stratoscopes were two balloon-borne astronomical telescopes which flew from the 1950s to the 1970s and observed in the optical and infrared regions of the spectrum. Both were controlled remotely from the ground.
Stratoscope I possessed a 12 inch (30.48 cm) mirror and was first flown in 1957. It was conceived by Martin Schwarzschild and built by the Perkin Elmer Corporation. A small secondary mirror focussed the image from the primary into a 35 mm movie camera, which captured the images on film. Schwarzschild used the telescope to study the turbulence and granulation in the Sun's photosphere.
Sputnik 1 was the first artificial Earth satellite. The Soviet Union launched it into an elliptical low Earth orbit on 4 October 1957. The density of the upper atmosphere could be deduced from its drag on the orbit, and the propagation of its radio signals gave information about the ionosphere. The scientist tasks to be carried out by a satellite included measuring the density of the atmosphere, its ion composition, corpuscular solar radiation, magnetic fields, cosmic rays, etc. But, the heavy scientific equipment was forgone in favour of a simple radio transmitter.
Regions
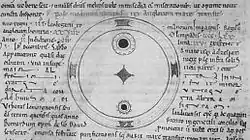
"Studies of stratigraphic data have suggested that the solar cycles have been active for hundreds of millions of years, if not longer; measuring varves in precambrian sedimentary rock has revealed repeating peaks in layer thickness, with a pattern repeating approximately every eleven years. It is possible that the early atmosphere on Earth was more sensitive to changes in solar radiation than today, so that greater glacial melting (and thicker sediment deposits) could have occurred during years with greater sunspot activity.[135] [136] This would presume annual layering; however, alternate explanations (diurnal) have also been proposed.[137]
Analysis of tree rings has revealed a detailed picture of past solar cycles: Dendrochronologically dated radiocarbon concentrations have allowed for a reconstruction of sunspot activity dating back 11,400 years, far beyond the four centuries of available, reliable records from direct solar observation.[138]
The earliest surviving record of sunspot observation dates from 364 BC, based on comments by Chinese astronomer Gan De in a star catalogue.[139] By 28 BC, Chinese astronomers were regularly recording sunspot observations in official imperial records.[140]
Astromathematics
Spherical triangles were studied by early Greek mathematicians such as Menelaus of Alexandria, who wrote a book on spherical triangles called Sphaerica and developed Menelaus' theorem.[141] E. S. Kennedy, however, points out that while it was possible in ancient mathematics to compute the magnitudes of a spherical figure, in principle, by use of the table of chords and Menelaus' theorem, the application of the theorem to spherical problems was very difficult in practice.[142]
Tycho Brahe remarks that the nature of understanding spherical triangles is so divine and elevated that it is not appropriate to extend its mysteries to everyone. (Diuinior et excellentior sit Triangulorum sphæricorum cognitio, quam fas sit eius mysteria omnibus propalare.)"
Physics
"The first recorded use of the word magnetohydrodynamics is by Hannes Alfvén in 1942:
- "At last some remarks are made about the transfer of momentum from the Sun to the planets, which is fundamental to the theory (§11). The importance of the magnetohydrodynamic waves in this respect are pointed out."[143]
Hypotheses
- As the placement or relationship of the Sun with the Earth probably hasn't changed substantially for millions of years, evidence for sunspot cycles or solar cycles should be available.
See also
- Radiation
- Radiation astronomy
- Radiation chemistry
- Radiation detectors
- Radiation geography
- Radiation telescopes
- Radiation satellites
References
- ↑ GS Hawkins (1966). Stonehenge Decoded. ISBN 978-0880291477.
- ↑ O. Neugebauer (January 1945). "The History of Ancient Astronomy Problems and Methods". Journal of Near Eastern Studies 4 (1): 1-38. http://www.jstor.org/stable/542323. Retrieved 2011-11-01.
- ↑ Charles H. Kahn (1970). "On early Greek astronomy". The Journal of Hellenic Studies 90: 99-116. http://www.jstor.org/stable/629756. Retrieved 2011-11-01.
- ↑ Herschel.
- ↑ Simon Schaffer (1980). "Herschel in Bedlam: Natural History and Stellar Astronomy". The British Journal for the History of Science 13 (03): 211-39. doi:10.1017/S0007087400018045. http://journals.cambridge.org/abstract_S0007087400018045. Retrieved 2011-11-01.
- 1 2 3 David Brown (2000). Cuneiform Monographs 18: Mesopotamian Planetary Astronomy-Astrology (PDF). Groningen: Styx Publications. pp. 113–20. Retrieved 2011-11-01.
- ↑ Results for "astronomy, In: Merriam-Webster Online. Retrieved 2007-06-20.
- ↑ B. Gardini (2011). "ESA strategy for human exploration and the Lunar Lander Mission". Memorie della Societa Astronomica Italiana 82: 422-9.
- ↑ Tai Wen-sai (21 February 1961). "PROBLEMS OF THE EVOLUTION OF HEAVENLY BODIE". Jen-Mln Jih-Pao: 7. http://www.dtic.mil/get-tr-doc/pdf?AD=ADA365913. Retrieved 2018-1-07.
- ↑ Hippolytus (Antipope); Francis Legge; Origen (1921). Philosophumena. 1. Original from Harvard University: Society for promoting Christian knowledge. Retrieved May 9, 2006.
- ↑ David W. Hughes (1999). "Book Review: The history and practice of ancient astronomy/Oxford U Press, 1998". Journal of the British Astronomical Association 109 (3): 164. http://adsabs.harvard.edu/full/1999JBAA..109..164H. Retrieved 2017-08-02.
- ↑ Everett C. Dolman (2005). Introduction: Realism and Geopolitics, In: Astropolitik: Classical Geopolitics in the Space Age. Southgate, London, England: Frank Cass Publishers. pp. 1–10. Retrieved 2017-08-02.
- ↑ Sam Kean (12 July 2010). The Disappearing Spoon: And Other True Tales of Madness, Love, and the History of the World from the Periodic Table of the Elements. Little, Brown. p. 400. Retrieved 2017-08-02.
- ↑ Morris Kline, Mathematical Thought from Ancient to Modern Times, p 299. Oxford University Press, 1972.
- ↑ D. Pacini (1912). "La radiazione penetrante alla superficie ed in seno alle acque". Il Nuovo Cimento, Series VI 3: 93–100. doi:10.1007/BF02957440.
- Translated and commented in A. de Angelis (2010). Penetrating Radiation at the Surface of and in Water.
- 1 2 3 Nobel Prize in Physics 1936 – Presentation Speech.
- ↑ V.F. Hess (1936). The Nobel Prize in Physics 1936. The Nobel Foundation. Retrieved 2010-02-11.
- ↑ V.F. Hess (1936). Unsolved Problems in Physics: Tasks for the Immediate Future in Cosmic Ray Studies. Nobel Lectures. The Nobel Foundation. Retrieved 2010-02-11.
- ↑ Ernest Rutherford. Retrieved 2012-08-16.
- ↑ Rutherford, E. (1920). "Bakerian Lecture. Nuclear Constitution of Atoms". Proceedings of the Royal Society A: Mathematical, Physical and Engineering Sciences 97 (686): 374. doi:10.1098/rspa.1920.0040.
- ↑ "V. A. Ambartsumian— a life in science". Astrophysics 51 (3): 280. 2008. doi:10.1007/s10511-008-9016-6. http://www.springerlink.com/content/ek2q156624661848/fulltext.pdf.
- ↑ Ambartsumian and Ivanenko (1930) "Об одном следствии теории дирака протонов и электронов" (On a Consequence of the Dirac Theory of Protons and Electrons), Доклады Академии Наук СССР (Doklady Akademii Nauk SSSR / Proceedings of the USSR Academy of Sciences) Ser. A, no. 6, pages 153-155. Available in Russian on-line.
- ↑ Bothe, W.; Becker, H. (1930). "Künstliche Erregung von Kern-γ-Strahlen". Zeitschrift für Physik 66 (5–6): 289. doi:10.1007/BF01390908.
- ↑ Becker, H.; Bothe, W. (1932). "Die in Bor und Beryllium erregten γ-Strahlen". Zeitschrift für Physik 76 (7–8): 421. doi:10.1007/BF01336726.
- ↑ Joliot-Curie Irène; Joliot Frédéric (1932). "Émission de protons de grande vitesse par les substances hydrogénées sous l'influence des rayons γ très pénétrants". Comptes Rendus 194: 273. http://visualiseur.bnf.fr/CadresFenetre?O=NUMM-3147&I=1236.
- ↑ Chadwick, J. (1933). "Bakerian Lecture. The Neutron". Proceedings of the Royal Society A: Mathematical, Physical and Engineering Sciences 142 (846): 1. doi:10.1098/rspa.1933.0152.
- ↑ Chadwick, James (1932). "Possible Existence of a Neutron". Nature 129 (3252): 312. doi:10.1038/129312a0.
- ↑ Wolfgang Pauli, In: Das Jahr 1932 Die Entdeckung des Neutrons. 6. 1985. p. 105. doi:10.1007/978-3-540-78801-0_3. ISBN 978-3-540-13609-5.
- ↑ Frank Close. Antimatter. Oxford University Press. pp. 50–52. ISBN 978-0-19-955016-6.
- 1 2 general chemistry. Taylor & Francis. p. 660. Retrieved 15 June 2011.
- ↑ Cowan, Eugene (1982). "The Picture That Was Not Reversed". Engineering & Science 46 (2): 6–28.
- ↑ Jagdish Mehra, Helmut Rechenberg (2000). The Historical Development of Quantum Theory, Volume 6: The Completion of. Quantum Mechanics 1926–1941. Springer. p. 804. ISBN 978-0-387-95175-1.
- ↑ Anderson, Carl D. (1933). "The Positive Electron". Physical Review 43 (6): 491–494. doi:10.1103/PhysRev.43.491.
- ↑ The Nobel Prize in Physics 1936. Retrieved 2010-01-21.
- ↑ K. Riesselmann (2007). "Logbook: Neutrino Invention". Symmetry Magazine 4 (2). http://www.symmetrymagazine.org/cms/?pid=1000450.
- ↑ K.-C. Wang (1942). "A Suggestion on the Detection of the Neutrino". Physical Review 61 (1–2): 97. doi:10.1103/PhysRev.61.97.
- ↑ C.L Cowan Jr.; F. Reines; F.B. Harrison; H.W. Kruse; A.D McGuire (1956). "Detection of the Free Neutrino: a Confirmation". Science 124 (3212): 103–4. doi:10.1126/science.124.3212.103. PMID 17796274.
- ↑ K. Winter (2000). Neutrino physics. Cambridge University Press. p. 38ff. ISBN 978-0-521-65003-8.
This source reproduces the 1956 paper. - ↑ The Nobel Prize in Physics 1995. The Nobel Foundation. Retrieved 29 June 2010.
- ↑ Christopher Bradly (24 October 2008). A New Two-Component Model of Spherical Galaxies with Dark Matter Halos (PDF). The University of Western Australia. p. 46. Retrieved 10 January 2018.
- ↑ Morrison, Philip (March 16, 1958). "On gamma-ray astronomy". Il Nuovo Cimento (1955-1965) 7 (6): 858–865. doi:10.1007/BF02745590. http://www.springerlink.com/content/3064g7043r2t77k5/. Retrieved 2010-11-14.
- ↑ Cosmic Rays Hunted Down: Physicists Closing in on Origin of Mysterious Particles. ScienceDaily. December 7, 2009. Retrieved 2010-11-14.
- 1 2 Barbara Mattson (October 18, 2007). OSO 1. Greenbelt, Maryland: NASA Goddard Space Flight Center. Retrieved 2012-06-10.
- ↑ J. Grygar (1985). "Gamma-radiation astronomy". Vesmir 64 (1): 10-12. https://inis.iaea.org/search/search.aspx?orig_q=RN:17020607. Retrieved 2018-1-07.
- ↑ "French, Soviets decide to launch Sigma space telescope". AFP Sci. (Paris): 11-12. 6 October 1983. http://adsabs.harvard.edu/abs/1983wers.rept....3.. Retrieved 2018-1-07.
- ↑ Virginia Trimble (1 January 1994). ""When one photon was a discovery, two was a spectrum, and three was the Rossi Prize", documents from the beginnings of gamma ray astronomy". AIP Conference Proceedings > 304 (1): 40-5. doi:10.1063/1.45679. https://cloudfront.escholarship.org/dist/prd/content/qt0xg3p605/qt0xg3p605.pdf. Retrieved 2018-1-10.
- 1 2 Bruce Hevly (1994). Gregory Good (ed.). Building a Washington Network for Atmospheric Research, In: The Earth, the Heavens, and the Carnegie Institution of Washington. Washington, DC: American Geophysical Union. pp. 143–8. ISBN 0-87590-279-0. Retrieved 2011-10-16.
- 1 2 3 Manuel Güdel (2004). "X-ray astronomy of stellar coronae". Astron Astrophys Rev 12 (2-3): 71-237. doi:10.1007/s00159-004-0023-2.
- ↑ Rolf Mewe (December 1996). "X-ray Spectroscopy of Stellar Coronae: History - Present - Future". Solar Physics 169 (2): 335-48. doi:10.1007/BF00190610.
- 1 2 T. R. Burnight (1949). "Soft X-radiation in the upper atmosphere". Physical Review A 76: 165.
- ↑ Pounds (1962). "A simple rocket-borne X-radiation monitor-its scope and results of an early flight". Monthly Notices of the Royal Astronomical Society 123: 347-57. http://adsabs.harvard.edu/full/1962MNRAS.123..347P. Retrieved 2011-10-16.
- ↑ Lyman, T. (1914). "Victor Schumann". Astrophysical Journal 38: 1–4. doi:10.1086/142050.
- ↑ Drake (1990, pp. 133–34)
- ↑ Sharratt (1994, pp. 1–2)
- 1 2 Antony Cooke (2005). Visual Astronomy Under Dark Skies: A New Approach to Observing Deep Space. London: Springer-Verlag. p. 180. ISBN 1852339012. Retrieved 2011-11-06.
- ↑ W. H. Wright (August 1927). "Photographs of Venus made by Infra-red and by Violet Light". Publications of the Astronomical Society of the Pacific 39 (230): 220-1. doi:10.1086/123718.
- ↑ F. J. Heyden; C. C. Kiess; Harriett K. Kiess (October 30, 1959). "Spectrum of Venus in the Violet and Near-Ultraviolet". Science 130 (3383): 1195. doi:10.1126/science.130.3383.1195. http://www.sciencemag.org/content/130/3383/1195.short. Retrieved 2012-06-01.
- 1 2 "Yerkes Observatory Finds Cyanogen in Spectrum of Halley's Comet". The New York Times. 8 February 1910. http://query.nytimes.com/gst/abstract.html?res=9407E4DF1430E233A2575BC0A9649C946196D6CF. Retrieved 15 November 2009.
- 1 2 3 "Ten Notable Apocalypses That (Obviously) Didn't Happen". Smithsonian magazine. 2009. http://www.smithsonianmag.com/history-archaeology/Ten-Notable-Apocalypses-That-Obviously-Didnt-Happen.html. Retrieved 14 November 2009.
- ↑ C. Y. Fan (September 1958). "Time Variation of the Intensity of Auroral Hydrogen Emission and the Magnetic Disturbance". The Astrophysical Journal 128 (9): 420-7. doi:10.1086/146556.
- ↑ Blue, Jennifer (November 9, 2009). Planet and Satellite Names and Discoverers. USGS. Retrieved 2010-01-13.
- ↑ Cruikshank, D. P.; Nelson R. M. (2007). Lopes, R. M. C.; Spencer, J. R. (eds.). A history of the exploration of Io, In: Io after Galileo. Springer-Praxis. pp. 5–33. ISBN 3-540-34681-3.
- ↑ Robert Grant Aitken (1961). The Binary Stars. Dover. p. 1.
- ↑ Hartkopf, W.; Mason, D. M. (2008). Sixth Catalog of Orbits of Visual Binaries. U.S. Naval Observatory.
- ↑ Herschel, J.F.W. (1847). Results of Astronomical Observations made during the years 1834,5,6,7,8 at the Cape of Good Hope; being the completion of a telescopic survey of the whole surface of the visible heavens, commenced in 1825. Smith, Elder and Co, London.
- ↑ Donald E. Osterbrock (May 2002). "Young Don Menzel's amazing adventures at Lick Observatory". Journal for the History of Astronomy 33 (111): 95-118.
- ↑ Herschel Discovers Infrared Light. Retrieved 2010-04-09.
- ↑ Rieke, George H. (2009). "History of infrared telescopes and astronomy". Experimental Astronomy 25 (1-3): 125–141. doi:10.1007/s10686-009-9148-7.
- ↑ J. Mofensen, Radar echoes from the moon, Electronics, vol. 19, pp. 92-98; April, 1946
- ↑ Z. Bay, "Reflection of microwaves from the moon," Hung. Acta Phys., vol. 1, pp. 1-22; April, 1946.
- ↑ Nelson Christensen; Renate Meyer; Adam Libson (2004). "A Metropolis–Hastings routine for estimating parameters from compact binary inspiral events with laser interferometric gravitational radiation data". CLASSICAL AND QUANTUM GRAVITY: 317–330. doi:10.1088/0264-9381/21/1/023. http://people.carleton.edu/~nchriste/CQG03.pdf. Retrieved 2018-1-07.
- ↑ Kenichi Ueda (2006). "New solid laser: Ceramic laser. From ultra stable laser to ultra high output laser". Kagaku (Tokyo) 76 (10): 1029-1034. https://inis.iaea.org/search/search.aspx?orig_q=RN:38009025. Retrieved 2018-1-07.
- ↑ H. M. Collins (1 June 1987). "Misunderstanding replication?". Social Science Information 26 (2): 451-459. doi:10.1177/053901887026002012. http://journals.sagepub.com/doi/abs/10.1177/053901887026002012?journalCode=ssia. Retrieved 2018-1-09.
- ↑ Gary W. Spetz (Spring 1981). "Reviewed Work: Sources of gravitational astronomy by Larry Smarr". The Journal of Mind and Behavior 2 (1): 105-111. http://www.jstor.org/stable/43852846. Retrieved 2018-1-09.
- 1 2 3 4 5 6 7 8 9 10 11 Jean Baptiste Joseph Delambre (1817). Histoire de l'astronomie ancienne. Paris: Courcier. p. 639. Retrieved 2012-01-13.
- 1 2 3 4 Jacob Ennis (August 1878). "Electricity and the Solar System". Astronomical Register 16: 255-6.
- ↑ Ludwig Oster; Kenelm W. Philip (January 1961). "Existence of Net Electric Charges on Stars". Nature 189 (4758): 43. doi:10.1038/189043a0.
- ↑ V. A. Bailey (January 1961). "Existence of Net Electric Charges on Stars". Nature 189 (4758): 43-4. doi:10.1038/189043b0.
- ↑ R. Krotkov; R. H. Dicke (June 1959). "Comparison between theory and observation for the outer planets". The Astronomical Journal 64 (1270): 157-62.
- 1 2 3 4 5 6 7 8 9 10 11 12 A. L. Cortie (December 1900). "Synopsis of Lecture on "The Solar Corona" by the Rev. A.L. Cortie to the Members of the North-Western Branch (Manchester) on 7th November 1900". Journal of the British Astronomical Association 11 (12): 77-8. http://adsabs.harvard.edu/cgi-bin/nph-data_query?bibcode=1900JBAA...11...77C&link_type=ARTICLE&db_key=AST&high=. Retrieved 2011-11-09.
- 1 2 3 Edison Pettit (July 1943). "The Properties of Solar Prominences as Related to Type". Astrophysical Journal 98 (7): 6-19. doi:10.1086/144539.
- 1 2 3 Edison Pettit (April 1945). "An Interactive Prominence". Publications of the Astronomical Society of the Pacific 57 (335): 97-9. doi:10.1086/125695.
- 1 2 3 4 5 6 Harold Zirin (March 1959). "Physical Conditions in Limb Flares and Active Prominences. II. a Remarkable Limb Flare, December 18, 1956". Astrophysical Journal 129 (3): 414-23. doi:10.1086/146633.
- 1 2 3 E. Walter Maunder (1903). "Spoerer's law of zones". The Observatory 26 (334): 329-30.
- 1 2 3 E. Walter Maunder; A. S. D. Maunder (June 1905). "The Solar Rotation Period from Greenwich Sun-spot Measures, 1879-1901". Monthly Notices of the Royal Astronomical Society 65 (8): 813-25.
- ↑ Bradley E. Schaefer (2007). "The Latitude and Epoch for the Origin of the Astronomical Lore in Mul.Apin". American Astronomical Society Meeting 210 #42.05 (American Astronomical Society) 38: 157.
- ↑ Hermann Hunger, David Pingree (1989). "MUL.APIN: An Astronomical Compendium in Cuneiform". Archiv für Orientforschung (Austria: Verlag Ferdinand Berger & Sohne Gesellschaft MBH) 24: 146.
- ↑ Staff (2008). MESSENGER: Mercury and Ancient Cultures. NASA JPL. Retrieved 2008-04-07.
- ↑ Immanuel Velikovsky (January 1965). Worlds in Collision. New York: Dell Publishing Co., Inc. p. 401. Retrieved 2012-01-13.
- ↑ Bartel Waerden (1974). Science awakening II: the birth of astronomy. Springer. p. 56. ISBN 9001931030. Retrieved 2011-01-10.
- ↑ M. E. Lefébure (November 1900). "The Zodiacal Light according to the Ancients". The Observatory, A Monthly Review of Astronomy 23 (298): 393-8. http://adsabs.harvard.edu/cgi-bin/nph-data_query?bibcode=1900Obs....23..393.&link_type=ARTICLE&db_key=AST&high=. Retrieved 2011-11-08.
- ↑ Marshack, Alexander (1991): The Roots of Civilization, Colonial Hill, Mount Kisco, NY.
- ↑ Brooks, A. S. and Smith, C. C. (1987): "Ishango revisited: new age determinations and cultural interpretations", The African Archaeological Review, 5 : 65–78.
- ↑ David Ewing Duncan (1998). The Calendar. Fourth Estate Ltd. pp. 10–11. ISBN 978-1-85702-721-1.
- ↑ A. Aaboe; J. P. Britton; J. A. Henderson; Otto Neugebauer; A. J. Sachs (1991). "Saros Cycle Dates and Related Babylonian Astronomical Texts". Transactions of the American Philosophical Society (American Philosophical Society) 81 (6): 1–75. doi:10.2307/1006543. "One comprises what we have called "Saros Cycle Texts," which give the months of eclipse possibilities arranged in consistent cycles of 223 months (or 18 years)."
- ↑ K. V. Sarma (2008). Helaine Selin (ed.). Astronomy in India, In: Encyclopaedia of the History of Science, Technology, and Medicine in Non-Western Cultures (2 ed.). Springer. pp. 317–321. ISBN 978-1-4020-4559-2.
- 1 2 Joseph Needham (1986). Science and Civilization in China, Volume III: Mathematics and the Sciences of the Heavens and Earth. Taipei: Caves Books. ISBN 0-521-05801-5.
- ↑ WD McKaig (1966). "Lunar Scientific Missions". SAE Technical Paper 660449 75 (A). doi:10.4271/660449. http://papers.sae.org/660449/. Retrieved 2018-1-07.
- ↑ B. Novakovic (2008). "Senenmut: An Ancient Egyptian Astronomer". Publications of the Astronomical Observatory of Belgrade 85: 19–23.
- ↑ Marshall Clagett (1989). Ancient Egyptian Science: Calendars, clocks, and astronomy, In: Ancient Egyptian Science. 2. DIANE Publishing. pp. 162–163. ISBN 0-87169-214-7.
- ↑ North, John David (2008). Cosmos: an illustrated history of astronomy and cosmology. University of Chicago Press. pp. 48–52. ISBN 0-226-59441-6.
- ↑ Swerdlow, Noel M. (1998). Periodicity and Variability of Synodic Phenomenon, In: The Babylonian theory of the planets. Princeton University Press. pp. 34–72. ISBN 0-691-01196-6.
- ↑ A. Sachs (May 2, 1974). "Babylonian Observational Astronomy". Philosophical Transactions of the Royal Society of London (Royal Society of London) 276 (1257): 43–50 (see p. 44). doi:10.1098/rsta.1974.0008.
- ↑ Eric Burgess (1982). By Jupiter: Odysseys to a Giant. New York: Columbia University Press. ISBN 0-231-05176-X.
- ↑ Rogers, J. H. (1998). "Origins of the ancient constellations: I. The Mesopotamian traditions". Journal of the British Astronomical Association, 108: 9–28.
- ↑ Saturn > Observing Saturn. National Maritime Museum. Retrieved 2007-07-06.
- ↑ A. Sachs (May 2, 1974). "Babylonian Observational Astronomy". Philosophical Transactions of the Royal Society of London (Royal Society of London) 276 (1257): 43–50 [45 & 48–9]. doi:10.1098/rsta.1974.0008.
- 1 2 The Binary Stars, Robert Grant Aitken, New York: Dover, 1964, p. 1.
- ↑ Vol. 1, part 1, p. 422, Almagestum Novum, Giovanni Battista Riccioli, Bononiae: Ex typographia haeredis Victorij Benatij, 1651.
- ↑ A New View of Mizar, Leos Ondra, accessed on line May 26, 2007.
- 1 2 3 D. K. Yeomans (1998). Great Comets in History. Jet Propulsion Laboratory. Retrieved 15 March 2007.
- ↑ D. A. Mendis (1988). "A Postencounter view of comets". Annual Review of Astronomy and Astrophysics 26 (1): 11–49. doi:10.1146/annurev.aa.26.090188.000303.
- ↑ Ian Ridpath (1985). Through the comet’s tail. Revised extracts from A Comet Called Halley by Ian Ridpath, published by Cambridge University Press in 1985. Retrieved 2011-06-19.
- ↑ Brian Nunnally (May 16, 2011). This Week in Science History: Halley’s Comet. pfizer: ThinkScience Now. Retrieved 2011-06-19.
- ↑ Interesting Facts About Comets. Universe Today. 2009. Retrieved 15 January 2009.
- ↑ Heber D. Curtis (June 1910). "Photographs of Halley's Comet made at the Lick Observatory". Publications of the Astronomical Society of the Pacific 22 (132): 117-30.
- ↑ M. P. Puiseux (July 1904). "Ancient and Modern Ideas about the Milky Way". The Observatory 27 (7): 271-4. http://adsabs.harvard.edu/cgi-bin/nph-data_query?bibcode=1904Obs....27..271P&link_type=ARTICLE&db_key=AST&high=4ebb0ab57b09516. Retrieved 2011-11-08.
- 1 2 Kepple, George Robert, Glen W. Sanner (1998). The Night Sky Observer's Guide, Volume 1. Willmann-Bell, Inc. p. 18. ISBN 0-943396-58-1.
- ↑ Sinclair, R.M. (2006). Todd W. Bostwick and Bryan Bates (ed.). The Nature of Archaeoastronomy, In: Viewing the Sky Through Past and Present Cultures; Selected Papers from the Oxford VII International Conference on Archaeoastronomy. Pueblo Grande Museum Anthropological Papers. 15. City of Phoenix Parks and Recreation Department. pp. 13–26. ISBN 1-882572-38-6.
- ↑ Ruggles, C.L.N. (2005). Ancient Astronomy. ABC-Clio. ISBN 1-85109-477-6.
- ↑ Ruggles, C.L.N. (1999). Astronomy in Prehistoric Britain and Ireland. Yale University Press. ISBN 0-300-07814-5.
- ↑
- ↑
- ↑ Betsy Maestro (2004). The Story of Clocks and Calendars. New York: HarperCollins. p. 48. ISBN 0-688-14548-5.
- ↑ Needham, Volume 3, p.171
- ↑ Needham, Volume 3, p.242
- ↑ B. L. van der Waerden (1974). "The Earliest Form of the Epicycle Theory". Journal for the History of Astronomy 5: 175-85.
- ↑ D. Koutsoyiannis; A. N. Angelakis (2003). Hydrologic and Hydraulic Science and Technology in Ancient Greece, In: Encyclopedia of Water Science (PDF). New York: Marcel Dekker, Inc. pp. 415–7. Retrieved 2011-10-26.
- 1 2 Giulio Magli (2009). When the method is lacking, In: Mysteries and Discoveries of Archaeoastronomy from Giza to Easter Island (PDF). Rome, Italy: Copernicus Books. pp. 97–116. doi:10.1007/978-0-387-76566-2_5. ISBN 978-0-387-76564-8. Retrieved 2011-10-15.
- ↑ DoD News Briefing, February 15, 1996 1:30 pm EST (from a [United States Department of Defense] DoD news briefing. Accessed 2008-06-21.)
- ↑ Francoise Micheau. [(Rashed & Morelon 1996, pp. 985–1007) The Scientific Institutions in the Medieval Near East]. pp. 992–3. (Rashed & Morelon 1996, pp. 985–1007).
- 1 2 3 4 Dimitris Plantzos (July 1997). "Crystals and Lenses in the Graeco-Roman World". American Journal of Archaeology 101 (3): 451-64. http://www.hist-arch.uoi.gr/prosopiko/plantzos/Crystals.pdf. Retrieved 2011-10-17.
- ↑ AH Tunnacliffe, JG Hirst (1996). Optics. Kent, England. pp. 233–7. ISBN 0-900099-15-1.
- 1 2 Winthrop W. Dolan (1975). A Choice of Sundials. S. Greene Press. p. 146. ISBN 0828902100. Retrieved 2012-10-24.
- ↑ Williams, G.E. (1985). "Solar affinity of sedimentary cycles in the late Precambrian Elatina Formation". Australian Journal of Physics 38: 1027–1043.
- ↑ Information, Reed Business (1981). "Digging down under for sunspots". New Scientist 91: 147. http://books.google.com/?id=4CpdD9YdMeoC&pg=PA147&lpg=PA147. Retrieved 2010-07-14.
- ↑ Williams GE (1990). "Precambrian Cyclic Rhythmites: Solar-Climatic or Tidal Signatures?". Philosophical Transactions of the Royal Society of London. Series A, Mathematical and Physical Sciences 330: 445. http://adsabs.harvard.edu/abs/1990RSPTA.330..445W.
- ↑ Solanki SK, Usoskin IG, Kromer B, Schüssler M, Beer J (October 2004). "Unusual activity of the Sun during recent decades compared to the previous 11,000 years". Nature 431 (7012): 1084–1087. doi:10.1038/nature02995. PMID 15510145. http://www.ncdc.noaa.gov/paleo/pubs/solanki2004/solanki2004.html.
- ↑ NRICH (University of Cambridge), In: Early Astronomy and the Beginnings of a Mathematical Science. 2007. Retrieved 2010-07-14.
- ↑ "The Observation of Sunspots". UNESCO Courier. 1988. http://archive.is/uKqG. Retrieved 2010-07-14.
- ↑ John J. O'Connor; Edmond F. Robertson. Menelaus of Alexandria. University of St Andrews.
- ↑ Kennedy, E. S. (1969). The History of Trigonometry, 31st Yearbook. National Council of Teachers of Mathematics, Washington DC. p. 337. (cf. Syed Nomanul Haq. The Indian and Persian background. p. 68., in Seyyed Hossein Nasr, Oliver Leaman (1996). History of Islamic Philosophy. Routledge. pp. 52–70. ISBN 0-415-13159-6.)
- ↑ Alfvén, H., "On the cosmogony of the solar system III", Stockholms Observatoriums Annaler, vol. 14, pp.9.1-9.29
Further reading
- M. E. Lefébure (November 1900). "The Zodiacal Light according to the Ancients". The Observatory, A Monthly Review of Astronomy 23 (298): 393-8. http://adsabs.harvard.edu/cgi-bin/nph-data_query?bibcode=1900Obs....23..393.&link_type=ARTICLE&db_key=AST&high=. Retrieved 2011-11-08.
External links
- African Journals Online
- Bing Advanced search
- Google Books
- Google scholar Advanced Scholar Search
- International Astronomical Union
- JSTOR
- Lycos search
- NASA's National Space Science Data Center
- NCBI All Databases Search
- Office of Scientific & Technical Information
- Questia - The Online Library of Books and Journals
- SAGE journals online
- The SAO/NASA Astrophysics Data System
- Scirus for scientific information only advanced search
- SIMBAD Astronomical Database
- Spacecraft Query at NASA
- SpringerLink
- Taylor & Francis Online
- WikiDoc The Living Textbook of Medicine
- Wiley Online Library Advanced Search
- Yahoo Advanced Web Search
{{History of science resources}}