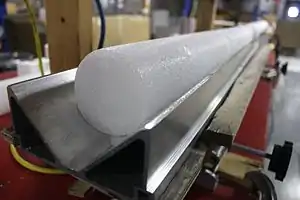
An ice core is a cylindrical sample of a rocky object consisting mostly of water ice. As shown in the image at the right, the long axis is in the direction of the coring into the object from its outer surface.
An ice core is taken with a hollow drill supported by a rig.
Coring
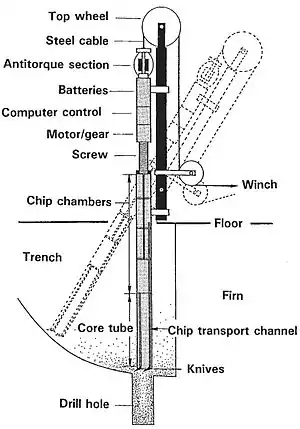
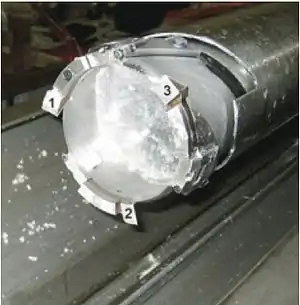

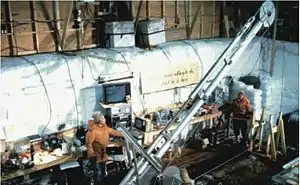

A core is collected by separating it from the surrounding material.
A diagram showing the components of a drill is at the right. The coring end of the drill has knives attached as shown in the image at the left. The drill above the knives is a hollow steel tube. The inner tube holds the ice core and the outer tube collects the cuttings or shavings from the action of the knives.
To power and stabilize the drill for coring, a rig such as in the photo at the second left is used with coring in progress.
The length of the drill barrel determines the maximum length of a core sample. Collection of a long core record requires many cycles of lowering a drill assembly, cutting a core 4–6 m in length, raising the assembly to the surface, emptying the core barrel, and preparing another assembly for drilling.
"Boring ice to depths in excess of about 300 meters requires a fluid with a density closely matched to that of ice to prevent lithostatic pressure from causing plastic collapse of the borehole; the latter frequently results in loss of the drilling equipment. The fluid, or mixture of fluids, must simultaneously satisfy criteria for density, low viscosity, frost resistance, as well as workplace safety and environmental compliance over both the short term (e.g., fire hazard and acute toxicity) and long term (chronic toxicity, local and global environmental degradation). The fluid must also satisfy other criteria, for example those stemming from the analytical methods employed on the ice core."[1]
"A number of different fluids and fluid combinations have been tried in the past. Since GISP2 (1990-1993) the US Polar Program has utilized a single-component fluid system, n-butyl acetate, but the toxicology, flammability, aggressive solvent nature, and longterm liabilities of n-butyl acetate raises serious questions about its continued application."[1]
"The European community, including the Russian program, has concentrated on the use of two-component drilling fluid consisting of low-density hydrocarbon base boosted to the density of ice by addition of halogenated-hydrocarbon (s.l.) densifier. Many of the proven densifier products are now considered too toxic, or are no longer available due to efforts to enforce the Montreal Protocol on ozone-depleting substances."[1]
In April 1998 on the Devon Ice Cap filtered lamp oil was used as a drilling fluid. In the Devon core it was observed that below about 150 m the stratigraphy was obscured by microfractures.[2]
When the drill barrel is filled, the barrel is removed from the rocky object and maneuvered, oriented, or tilted, as in the third image at the left, so that the ice core may be released, being performed in the image on the lowest left.
Contamination
Some contamination has been detected in ice cores.
The levels of lead on the outside of ice cores is much higher than on the inside.[3] In ice from the Vostok core (Antarctica), the outer portion of the cores have up to 3 and 2 orders of magnitude higher bacterial density and dissolved organic carbon than the inner portion of the cores, respectively, as a result of drilling and handling.[4]
Ice relaxation
Deep ice is under great pressure. When brought to the surface, there is a drastic change in pressure. Due to the internal pressure and varying composition, particularly bubbles, sometimes cores are very brittle and can break or shatter during handling. At Dome C, the first 1000 m were brittle ice. Siple dome encountered it from 400 to 1000 m. It has been found that allowing ice cores to rest for some time (sometimes for a year) makes them become much less brittle.
Decompression causes significant volume expansion (called relaxation) due to microcracking and the exsolving of clathrate hydrate (enclathratized) gases.[5]
Relaxation may last for months.[6] During this time, ice cores are stored below -10 °C to prevent cracking due to expansion at higher temperatures. At drilling sites, a relaxation area is often built within existing ice at a depth which allows ice core storage at temperatures below -20 °C.
It has been observed that the internal structure of ice undergoes distinct changes during relaxation. Changes include much more pronounced cloudy bands and much higher density of "white patches" and bubbles.[7]
Several techniques have been examined. Cores obtained by hot water drilling at Siple Dome in 1997–1998 underwent appreciably more relaxation than cores obtained with the PICO electro-mechanical drill. In addition, the fact that cores were allowed to remain at the surface at elevated temperature for several days likely promoted the onset of rapid relaxation.[8]
Theoretical ice cores
Def. a part of anything taken or presented for inspection, or shown as evidence of the quality of the whole is called a sample.
Def. a cylindrical sample of rock or other materials is called a core.
Def. "a core sample drilled from the accumulation of snow and ice over many years that have recrystallized and have trapped air bubbles from previous time periods, the composition of which can be used to reconstruct past climates and climate change; typically removed from an ice sheet (Antarctica and Greenland) or from high mountain glaciers elsewhere"[9] is called an ice core.
Electromagnetics
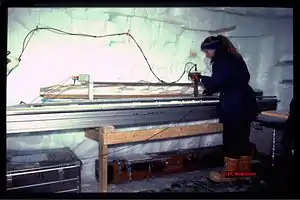
"Continuous measurements made in the field included dielectric profiling and electrical conductivity (related to the concentrations of neutral salts and acid)."[10]
Core processing
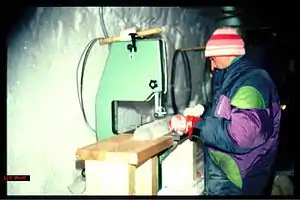
Modern practice is to ensure that cores remain uncontaminated, since they are analysed for trace quantities of chemicals and isotopes. They are sealed in plastic bags after drilling and analysed in clean rooms.
The core is carefully extruded from the barrel; often facilities are designed to accommodate the entire length of the core on a horizontal surface. Drilling fluid will be cleaned off before the core is cut into 1-2 meter sections. Various measurements may be taken during preliminary core processing.
Current practices to avoid contamination of ice include:
- Keeping ice well below the freezing point.
- At Greenland and Antarctic sites, temperature is maintained by having storage and work areas under the snow/ice surface.
- At GISP2, cores were never allowed to rise above -15 °C, partly to prevent microcracks from forming and allowing present-day air to contaminate the fossil air trapped in the ice fabric, and partly to inhibit recrystallization of the ice structure.
- Wearing special clean suits over cold weather clothing.
- Mittens or gloves.
- Filtered respirators.
- Plastic bags, often polyethylene, around ice cores. Some drill barrels include a liner.
- Proper cleaning of tools and laboratory equipment.
- Use of laminar-flow bench to isolate core from room particulates.
For shipping, cores are packed in styrofoam boxes protected by shock absorbing bubble-wrap.
Due to the many types of analysis done on core samples, sections of the core are scheduled for specific uses. After the core is ready for further analysis, each section is cut as required for tests. Some testing is done on site, other study will be done later, and a significant fraction of each core segment is reserved for archival storage for future needs.
Projects have used different core-processing strategies. Some projects have only done studies of physical properties in the field, while others have done significantly more study in the field. These differences are reflected in the core processing facilities.
Storage and transportation
Ice cores are typically stored and transported in refrigerated ISO container systems. Due to the high value and the temperature-sensitive nature of the ice core samples, container systems with primary and back-up refrigeration units and generator sets are often used. Known as a Refrigerated container, or Redundant Container System in the industry, the refrigeration unit and generator set automatically switches to its back-up in the case of a loss of performance or power to provide the ultimate peace of mind when shipping this valuable cargo.
Petrology
Def. the study of the origin, composition and structure of rock or rocky objects is called petrology.
"The Camp Century ice drilling continued till the summer of 1966, when the drill reached bedrock at a depth of 1390 metres. The deepest 25 metres of ice core consisted of silty ice containing bottom material of increasing coarseness downward from dust to small and larger stones. This indicated that the deepest ice had been in contact with the bedrock farther upstream from Camp Century."[11]
Petrochemistry
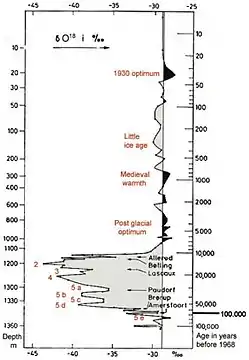
Many rocky objects are composed of oxide minerals. Oxygen has three known stable isotopes: 16O, 17O, and 18O.
"The stable isotopic compositions of low-mass (light) elements such as oxygen, hydrogen, [helium, lithium, beryllium, boron,] carbon, nitrogen, [fluorine, neon, sodium, magnesium, aluminum, silicon, phosphorus,] and sulfur are normally reported as "delta" ([δ]) values in parts per thousand (denoted as ‰ [per mille]) enrichments or depletions relative to a standard of known composition."[12]
For 18O to 16O:
The "ratio [by convention is] of the heavy to light isotope in the sample or standard."[12]
"Various isotope standards are used for reporting isotopic compositions; the compositions of each of the standards have been defined as 0‰. Stable oxygen and hydrogen isotopic ratios are normally reported relative to the SMOW standard ("Standard Mean Ocean Water" (Craig, 1961b)) or the virtually equivalent VSMOW (Vienna-SMOW) standard. Carbon stable isotope ratios are reported relative to the PDB (for Pee Dee Belemnite) or the equivalent VPDB (Vienna PDB) standard. The oxygen stable isotope ratios of carbonates are commonly reported relative to PDB or VPDB, also. Sulfur and nitrogen isotopes are reported relative to CDT (for Cañon Diablo Troilite) and AIR (for atmospheric air), respectively."[12]
"Ordinary water consists of slightly different kinds of so-called isotopic water molecules of equal chemical properties but different masses: a light one (H2O16), which occurs most frequently by far in natural waters, and quite a few heavier ones, of which the H2O18 and the [deuterium, D) HDO16 components occur in concentrations of approximately 2000 and 320 ppm (parts per million) water molecules, respectively. Due to slightly different vapour pressures and rates of reaction, the concentrations of the isotopic components change somewhat during phase-shifts in the natural water cycle".[11]
Ice "cores contain a wealth of information on past climates in the form of a great number of parameters, of which some are listed below:"[11]
- Concentrations of the oxygen-18 and deuterium components of water in the ice give information about the cloud temperature and precipitation at the time of deposition,
- the content of air in bubbles reveals the altitude of the then ice surface,
- the concentrations of carbon dioxide and methane in the air bubbles tell about the greenhouse effect in past atmospheres,
- the chemical composition of the ice itself gives information about other aspects of the chemistry in past atmospheres,
- dust and calcium concentration tell about the violence and frequency of the storms that carried dust from ice free areas to the inland ice, and
- the acidity of the ice indicates the fall-out of volcanic acids and thereby past volcanic activity.
Inclusions
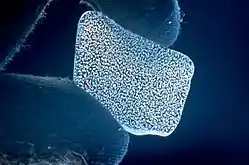

"A sliver of Antarctic ice [in the image at the right reveals] the myriad enclosed tiny bubbles of air. Air bubbles trapped in ice hundreds or even thousands of years ago are providing vital information about past levels of greenhouse gases in the Earth's atmosphere."[13] The image at the left shows the crystals and air bubbles in polarized light which varies with grain orientation.
"The only visible inclusions in glacier ice are the air bubbles, particularly as far as 14C, 10Be, and the greenhouse gases CO2 and methane are concerned [...]. The invisible inclusions, micro particles and chemical impurities, had potential to reveal other interesting facets of the environment of the past".[11]
Invisible "inclusions in the ice, especially dust and strong acids [...] are deposited with the snow. During interglacial time most of the dust comes from spring-dry areas in North America, and most of the strong acids always originate from volcanic eruptions in the northern hemisphere."[11]
A "Coulter counter [...] measures the concentration and size distribution of microscopic dust particles in the ice, which render information about the origin of the dust and the frequency and strength of the storms that bring it to Greenland."[11]
The "Crête core [contains] the fall-out of insoluble dust particles from the continents tops in early summer. Therefore, a continuous profile of the dust concentration along an ice core would allow a year by year dating by counting summer maxima downward from the surface, just like counting δ-maxima. Counting dust maxima may even extend the dating further back in time, because dust does not diffuse like the isotopic components of water"[11]
"Greenland ice deposited through the glaciation contains up to 100 times more dust than post-glacial ice. The summer maxima drown more or less in the high background values that are caused partly by dryer and more stormy climate then, partly by vast areas north of Siberia being drained due to lowering of the sea level, which creates a most efficient source of dust. In contrast, the dust counting method works also on glacial ice in Antarctica, where the air has always contained very little dust. There is simply no large ice-free land areas nearby that may serve as sources of dust."[11]
A "method for detecting great volcanic eruptions in the past [included] a series of conventional acidity (pH) measurements on ice samples deposited about A.D. 1783, when the Icelandic volcano Laki had a giant eruption. As expected, the 1783 layer was highly acid owing to fall-out of sulphuric acid. The volcano emitted sulphur dioxide (SO2) into the higher atmosphere, where it was quickly converted into tiny droplets of diluted sulphuric acid. Soon after they have fallen down into the lower atmosphere they are washed out by precipitation".[11]
Geochronology

Shallow cores, or the upper parts of cores in high-accumulation areas, can be dated exactly by counting individual layers, each representing a year. These layers may be visible, related to the nature of the ice; or they may be chemical, related to differential transport in different seasons; or they may be isotopic, reflecting the annual temperature signal (for example, snow from colder periods has less of the heavier isotopes of H and O). Deeper into the core the layers thin out due to ice flow and high pressure and eventually individual years cannot be distinguished. It may be possible to identify events such as nuclear bomb atmospheric testing's radioisotope layers in the upper levels, and ash layers corresponding to known volcanic eruptions. Volcanic eruptions may be detected by visible ash layers, acidic chemistry, or electrical resistance change. Some composition changes are detected by high-resolution scans of electrical resistance. Lower down the ages are reconstructed by modeling accumulation rate variations and ice flow.
Dating is a difficult task. Five different dating methods have been used for Vostok cores, with differences such as 300 years per meter at 100 m depth, 600yr/m at 200 m, 7000yr/m at 400 m, 5000yr/m at 800 m, 6000yr/m at 1600 m, and 5000yr/m at 1934 m.[14]
Different dating methods makes comparison and interpretation difficult. Matching peaks by visual examination of Moulton and Vostok ice cores suggests a time difference of about 10,000 years but proper interpretation requires knowing the reasons for the differences.[15]
Stratigraphy
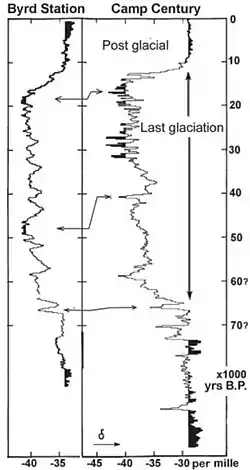
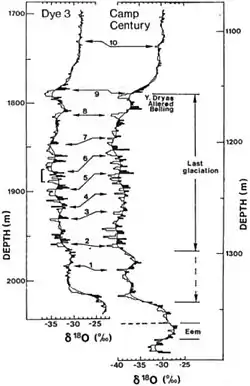
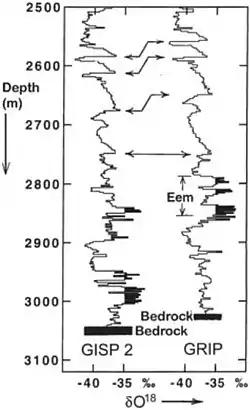
"The time scale to the right [in the diagram at the right] gives calculated ages in millennia. It has later been changed, particularly beyond 60.000 years. The double arrows show probable simultaneous events. In the coldest part of the glaciation, the climate obviously varied much more violently in Greenland than in Antarctica, probably due to strongly shifting ice cover in the North Atlantic Ocean. Note also the difference at the termination of the glaciation."[11]
An "ice dating method based on the radioactive 36Cl/10Be ratio [ref.11.8 has been applied to Dye 3. The] hydrogen peroxide concentration varies seasonally [ref.11.9] and may thus be used as an additional way of annual layer identification [The image at the left] shows δ-profiles along the deepest c.300 m, of the surface to bedrock ice cores from Dye 3 and Camp Century. The 10 double arrows point at common features suggesting layers of simultaneous deposition. Down to arrow No. 1 the two records are obviously similar indicating that the abrupt δ-shifts during late glacial time are outcomes of events common for the entire ice cap. Below arrow No. 1, however, the two records do not agree in detail. Probably, the Dye 3 core does not reach continuously as far back in time (90,000 years at most) as the Camp Century core, which is not surprising in view of the hilly bedrock upstream. The very deepest part may be of Eemian origin, though, i.e. from the last interglaciation some 125,000 years ago, but the stratigraphy is hardly undisturbed."[11]
"Down to a depth of 2750m the two profiles [in the figure at the lower right] are essentially identical, but they are different in ice from the Eem period. The layer sequence is disturbed in the GISP2 core. Is this also the case for the GRIP core?"[11]
Paleoclimatology
"We didn't realise 15 or 20 years ago that ice coring would become such a powerful technique. The biggest impact of ice cores on our understanding of climate change is the detail we've been able to see. We've also been able to confirm the link between our climate and changes in the orbit of the Sun. But perhaps the most significant thing ice cores have told us is that greenhouse gases are so well-related to climate, and that at no point in the last three-quarters of a million years have carbon dioxide and methane reached anywhere near the levels they are at today."[16]
"Given that the main driving force for long-term climate change is the orbit round the Sun, it's not obvious that the Earth's orbit has changed enough to cause this 40,000 year cycle to shift to a 100,000 year cycle. At the moment the change is difficult to understand, but we hope that a 1.4 million year ice core will help us."[16]
Ice cores contain an abundance of information about climate. Inclusions in the snow of each year remain in the ice, such as wind-blown dust, ash, bubbles of atmospheric gas and radioactive substances. The variety of climatic proxies is greater than in any other natural recorder of climate, such as tree rings or sediment layers. These include (proxies for) temperature, ocean volume, precipitation, chemistry and gas composition of the lower atmosphere, volcanic eruptions, solar variability, sea-surface productivity, desert extent and forest fires.
An ice core from the right site can be used to reconstruct an uninterrupted and detailed climate record extending over hundreds of thousands of years, providing information on a wide variety of aspects of climate at each point in time. It is the simultaneity of these properties recorded in the ice that makes ice cores such a powerful tool in paleoclimate research.
As porous snow consolidates into ice, the air within it is trapped in bubbles in the ice. This process continuously preserves samples of the atmosphere.[17]
In order to retrieve these natural samples the ice is ground at low temperatures, allowing the trapped air to escape. It is then condensed for analysis by gas chromatography or mass spectrometry, revealing gas concentrations and their isotopic composition respectively. Apart from the intrinsic importance of knowing relative gas concentrations (e.g. to estimate the extent of greenhouse warming), their isotopic composition can provide information on the sources of gases. For example CO2 from fossil-fuel or biomass burning is relatively depleted in 13C. See Friedli et al., 1986.
Dating the air with respect to the ice it is trapped in is problematic. The consolidation of snow to ice necessary to trap the air takes place at depth (the 'trapping depth') once the pressure of overlying snow is great enough. Since air can freely diffuse from the overlying atmosphere throughout the upper unconsolidated layer (the 'firn'), trapped air is younger than the ice surrounding it.
Trapping depth varies with climatic conditions, so the air-ice age difference could vary between 2500 and 6000 years (Barnola et al., 1991). However, air from the overlying atmosphere may not mix uniformly throughout the firn (Battle et al., 1986) as earlier assumed, meaning estimates of the air-ice age difference could be less than imagined. Either way, this age difference is a critical uncertainty in dating ice-core air samples. In addition, gas movement would be different for various gases; for example, larger molecules would be unable to move at a different depth than smaller molecules so the ages of gases at a certain depth may be different. Some gases also have characteristics which affect their inclusion, such as helium not being trapped because it is soluble in ice.
In Law Dome ice cores, the trapping depth at DE08 was found to be 72 m where the age of the ice is 40±1 years; at DE08-2 to be 72 m depth and 40 years; and at DSS to be 66 m depth and 68 years.[18]
Materials
Many materials can appear in an ice core. Layers can be measured in several ways to identify changes in composition. Small meteorites may be embedded in the ice. Volcanic eruptions leave identifiable ash layers. Dust in the core can be linked to increased desert area or wind speed.
Isotopic analysis of the ice in the core can be linked to temperature and global sea level variations. Analysis of the air contained in bubbles in the ice can reveal the palaeocomposition of the atmosphere, in particular CO2 variations. There are great problems relating the dating of the included bubbles to the dating of the ice, since the bubbles only slowly "close off" after the ice has been deposited. Nonetheless, recent work has tended to show that during deglaciations CO2 increases lag temperature increases by 600 +/- 400 years.[19] Beryllium-10 concentrations are linked to cosmic ray intensity which can be a proxy for solar strength.
There may be an association between atmospheric nitrates in ice and solar activity. However, recently it was discovered that sunlight triggers chemical changes within top levels of firn which significantly alter the pore air composition. This raises levels of formaldehyde and NOx. Although the remaining levels of nitrates may indeed be indicators of solar activity, there is ongoing investigation of resulting and related effects of effects upon ice core data.[20][21]
Geography
Ice cores have been taken from many locations around the world. Major efforts have taken place on Greenland and Antarctica.
Sites on Greenland are more susceptible to snow melt than those in Antarctica. In the Antarctic, areas around the Antarctic Peninsula and seas to the west have been found to be affected by El Niño ENSO effects. Both of these characteristics have been used to study such variations over long spans of time.[22]
Penny Ice Cap
The Penny Ice Cap is on Baffin Island, Canada, at 67° 15'N, 65° 45'W, 1900 masl.
"Snowpits were excavated in 1994 and 1995."[23]
"The 333.78m. P95 core was drilled to the bed in April-May 1995. The 89m. P95.2 core was drilled adjacent to the P95 core (2.5 m. away) for evaluation of noise in the record. The d18O correlation between the two cores is .80."[23]
"The 177.91m. P96 core also reached the bed. It is located approximately 16 km. from P95. The P95-P96 correlation of d18O on 40-year segments is .3 to .5."[23]
"The P95 core was dated back to 7900 yr ago (319 m) by spectral analysis of the electrical conductivity record which tracks seasonal chemical variations (Fisher et al. 1998; Grumet et al., 1998. Additional time control was provided by conductivity and sulfate peaks related major volcanic eruptions. Beyond 7900 yr ago, the depth-age curve was adjusted to match the end of the Younger Dryas-Holocene transition (326 m) dated at 11,550 +/- 70 yr ago in Greenland ice-core records. Age values for the P95 microparticle record are expressed in years before A.D. 2000 (b2k)."[23]
Greenland
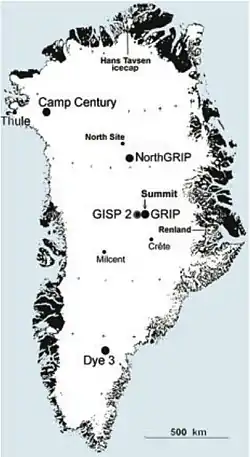
In addition to many of the ice core drilling sites on Greenland in the image at the right, there are the separate ice caps on Hans Tavsen in Peary Land way to the north and Renland in the east.
Station Eismitte
Alfred "Wegener set out for his famous expedition 1929-30, the crux of which became the wintering station Eismitte in Central Greenland 500 km from the west coast."[11]
"The ice thickness was measured at c. 2500 m by bursting dynamite on the surface and picking up the echoes from the bedrock. Bursting experiments performed next summer in the marginal zone showed that Greenland is like a bowl filled with ice, which slowly flows through chips in the edge ending up as icebergs or meltwater plunging into the sea."[11]
Eismitte means Ice-Center in German, and the campsite was located 402 kilometers (250 miles) from the coast at an estimated altitude of 3,000 meters (9,843 feet).
As a member of the Alfred Wegener Expedition to Eismitte in central Greenland from July 1930 to August 1931, Ernst Sorge hand-dug a 15 m deep pit adjacent to his beneath-the-surface snow cave. Sorge was the first to systematically and quantitatively study the near-surface snow/firn strata from inside his pit. His research validated the feasibility of measuring the preserved annual snow accumulation cycles, like measuring frozen precipitation in a rain gauge.[24]
Camp VI
During 1948/51 members of Expeditions Polaires Francaises (EPF) led by Paul Emile Victor reported boring two holes to depths of 125 m at Camp VI and 150 m at Eismitte.[25]
Camp VI is in the western part of Greenland on the EPF-EGIG line at an elevation of 1598 masl.
Station Centrale was not far from Alfred Wegener's station Eismitte.[11] Centrale is on a line between Milcent (70°18’N 45°35’W, 2410 masl) and Crête (71°7’N 37°19’W), at about (70°43'N 41°26'W), whereas Eismitte is at (71°10’N 39°56’W, ~3000 masl).
Site 2
In 1956, pre-International Geophysical Year (IGY) of 1957-58, a 10 cm diameter core using a rotary mechanical drill (US) to 305 m was recovered.[24]
A second 10 cm diameter core was recovered in 1957 by the same drill rig to 411 m. A commercially modified, mechanical-rotary Failing-1500 rock-coring rig was used, fitted with special ice cutting bits.[24]
Camp Century
Three cores were attempted at Camp Century in 1961, 1962, and again in 1963. The third hole was started in 1963 and reached 264 m. The 1963 hole was re-entered using the thermal drill (US) in 1964 and extended to 535 m. In mid-1965 the thermal drill was replaced with an electro-mechanical drill, 9.1 cm diameter, that reached the base of the ice sheet in July 1966 at 1387 m. The Camp Century, Greenland, (77°10’N 61°8’W, 1885 masl) ice core (cored from 1963-1966) is 1390 m deep and contains climatic oscillations with periods of 120, 940, and 13,000 years.[26]
Another core in 1977 was drilled at Camp Century using a Shallow (Dane) drill type, 7.6 cm diameter, to 100 m.
North Site
The Greenland Ice Sheet Program (GISP) took short-depth corings at several locations on the Greenland ice sheet. The corings began after the formation of the GISP consortium in 1972 and continued until the end of Dye 3 in 1981.
"In 1972, [...] with a SIPRE auger [7.6 cm diameter] at a location [...] called North Site [75°46’N 42°27’W], 500 km north of the EGIG line, 2870m a.s.l. [they] recovered a nice core to 25m depth [...] The δ-analyses indicated an accumulation rate of only 16 cm of ice per year, and already at a depth of 6-7m the diffusion had obliterated some of the seasonal cycles".[11]
North Central
The first core at North Central (74°37’N 39°36’W) was drilled in 1972 using a Shallow (Dane) drill type, 7.6 cm diameter to 100 m.
Milcent
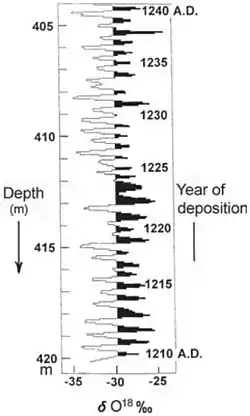
In 1973, a "398m [12.4 cm in diameter core was drilled at Milcent (70.3°N, 44.6°W, 2410 masl) with a] thermo-drill, and a mean accumulation of 56 cm of ice per year left the most beautiful annual δ-cycles we have seen anywhere (Fig. 8.3). It was quite easy to date the entire core back to A.D. 1177 with a ±2 years accuracy. But there was a general tendency toward decreasing δ’s downwards in the core, undoubtedly because deeper ice is deposited further inland and therefore at lower temperature."[11]
"The first core drilled at Station Milcent in central Greenland covers the past 780 years."[27]
Crête
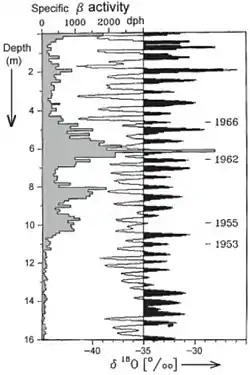

"In 1974, [in central Greenland (71°7’N 37°19’W)] thermo-drilling [7.6 cm diameter core] took place at Crête in the coldest area of the inland ice (annual mean surface temperature -32 ̊C). The mean accumulation rate 28 cm of ice per year was marginal for the survival of the annual δ-cycles [image at the right], but a model was developed for the diffusion re-establishing the almost obliterated cycles – we called the technique for “reversed diffusion” [ref. 8.1]. Annual cycle counting showed that the oldest layer in the 404 m long core was deposited in A.D. 534."[11]
"The Crête core was drilled in central Greenland (1974) and reached a depth of 404.64 meters, extending back only about fifteen centuries.[28]
In the diagram at the right β-radiation in dose per hour (dph) and δ measurements along the upper 16 m of the Crête core from 1974 are aligned to depth.
"The seasonal cycles in the δ curve to the right have decreasing amplitude downward, because the diffusion in the porous firn tends toward elimination of all δ-gradients. However, the cycles are distinct enough for exact dating back to 1942. The grey shaded curve shows the specific β-radioactivity profile: There is no trace of fall-out from the nuclear bombs in 1945, but the first hydrogen bombs in 1953-55 caused considerable radioactive fall-out on the inland ice, and so did the test series in 1958-59 and in the early 1960’es."[11]
The Crête 1984 ice cores consist of 8 short cores drilled in the 1984-85 field season as part of the post-GISP campaigns. Glaciological investigations were carried out in the field at eight core sites (A-H).[29]
The second diagram at the right "shows the mean acidity of each of the 1440 annual layers in the Crête core identified by the δ-method [...]. Many known, and far more unknown volcanic eruptions in the northern hemisphere are revealed in this record. Observe that the acidity does not directly indicate the magnitude of eruptions. Those in low latitudes do not appear so strongly as similar eruption in the nearby Iceland. Notice in [the diagram] the moderate volcanic activity in the medieval period 850 to 1250 and in the 20’th century, both warm periods, and the intense volcanic activity in “The Little Ice Age” 1350-1700."[11]
Dye 2
Drilling with a Shallow (Swiss) drill type at Dye 2 (66°23’N 46°11’W, 2338 masl) began in 1973. The core was 7.6 cm in diameter to a depth of 50 m. A second core to 101 m was 10.2 cm in diameter was drilled in 1974. An additional core at Dye 2 was drilled in 1977 using a Shallow (US) drill type, 7.6 cm diameter, to 84 m.
Summit
The camp is located approximately 360 km from the east coast and 500 km from the west coast of Greenland at (Saattut, Uummannaq), and 200 km NNE of the historical ice sheet camp Eismitte. The closest town is Ittoqqortoormiit, 460 km ESE of the station. The station however is not part of Sermersooq municipality, but falls within the bounds of the Northeast Greenland National Park.
An initial core at Summit (71°17’N 37°56’W, 3212 masl) using a Shallow (Swiss) drill type was 7.6 cm in diameter for 31 m in 1974. Summit Camp, also Summit Station, is a year-round research station on the apex of the Greenland Ice Sheet. Its coordinates are variable, since the ice is moving. The coordinates provided here (72°34’45”N 38°27’26”W, 3212 masl) are as of 2006.
South Dome
The first core at South Dome (63°33’N 44°36’W, 2850 masl) used a Shallow (Swiss) drill type for a 7.6 cm diameter core to 80 m in 1975.
Hans Tausen (or Hans Tavsen)
The first GISP core drilled at Hans Tausen (82°30’N 38°20’W, 1270 masl) was in 1975 using a Shallow (Swiss) drill type, 7.6 cm diameter core to 60 m. The second core at Hans Tausen was drilled in 1976 using a Shallow (Dane) drill type, 7.6 cm diameter to 50 m. The drilling team reported that the drill was stuck in the drill hole and lost.
"The Swiss drill was used on the Danish-Swiss expedition [the first GISP core here in] 1975 to the Hans Tavsen ice cap in Peary Land, the world’s northernmost ice cap [...]. From the very start it was evident that there was considerable surface melting in the summer time, in spite of Hans Tavsen being a neighbour to the North Pole. Nevertheless, the location was interesting, because it lies close to the Arctic Ocean, from where Hans Tavsen undoubtedly receives most of its snow, contrary to more southerly regions of the inland ice."[11]
"The Hans Tausen ice cap in Peary Land was [drilled again] by a forerunner of a new deep drill that reached bedrock 325 m below the surface. [...] The ice core contained distinct melt layers all the way to bedrock indicating that, contrary to Renland, Hans Tavsen contains no ice from the glaciation. Thus, the world’s northernmost ice cap melted away during the post-glacial climatic optimum and was rebuilt when the climate got colder some 4000 years ago [-4000 b2k].[11]
Camp III
The first core at Camp III (69°43’N 50°8’W) was drilled in 1977 using a Shallow (Swiss) drill type, 7.6 cm, to 49 m. The last core at Camp III was drilled in 1978 using a Shallow (Swiss) drill type, 7.6 cm diameter, 80 m depth.
Dye 3
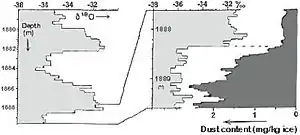

The Greenland Ice Sheet Project (GISP) including Dye 3 was a decade-long project to drill 20[30] ice cores in Greenland.
The Greenland Dye 3 1979 core is not completely intact and is not undamaged.
“Below 600 m, the ice became brittle with increasing depth and badly fractured between 800 and 1,200 m. The physical property of the core progressively improved and below ~1,400 m was of excellent quality.”[31]
“The deep ice core drilling terminated in August 1981. The ice core is 2035 m long and has a diameter of 10 cm. It was drilled with less than 6° deviation from vertical, and less than 2 m is missing. The deepest 22 m consists of silty ice with an increasing concentration of pebbles downward. In the depth interval 800 to 1400 m the ice was extremely brittle, and even careful handling unavoidably damaged this part of the core, but the rest of the core is in good to excellent condition.”[32]
The depth interval 800 to 1400 m would be a period approximately from about two thousand years ago to about five or six thousand years ago.[33]
Melting has been commonplace throughout the Holocene. Summer melting is usually the rule at Dye 3, and there is occasional melting even in north Greenland. All of these meltings disturb the clarity of the annual record to some degree.
“An exceptionally warm spell can produce features which extend downwards by percolation, along isolated channels, into the snow of several previous years. This can happen in regions which generally have little or no melting at the snow surface as exemplified during mid July 1954 in north-west Greenland4. Such an event could lead to the conclusion that two or three successive years had abnormally warm summers, whereas all the icing formed during a single period which lasted for several days. The location where melt features will have the greatest climactic significance is high in the percolation facies where summer melting is common but deep percolation is minimal4. Dye 3 in southern Greenland (65°11’N; 43°50’W) is such a location.”[34]
The brittle zone mentioned above corresponds in Dye 3 1979 with the steady state grain size (crystal size) from ~637 - ~1737 m depth range. This is also the Holocene climatic optimum period.
"The left part of [the figure on the upper right] shows two typical δ shifts measured through 111⁄2m or ca.4000 years of the Dye 3 core deposited some 25,000-30,000 years ago. The two abrupt δ leaps correspond to temperature increases of 10-12°C in less than a century. However, the subsequent cooling went off stepwise through much longer periods of the order of a millennium. [...] On the right: The eldest of the two is shown in detail and compared with the dust concentration changes in the same two metres of ice."[32]
In the figure at the lower right, the "section to the left shows the δ-profile along 150m of the deep ice core from Dye 3. The 1700 to 1850m depth interval spans most of the Pleistocene to Pre-Boreal transition, including the Bølling/Allerød – Younger Dryas oscillation. In the middle: Detailed δ-record through the Younger Dryas to Pre-Boreal transition, during which the South Greenland temperature increased by 15°C in 50 years. To the right: Deuterium excess and dust concentration shifted to lower levels in less than 20 years."[32]
Renland
In "1985, when [the final version of “the Rolls Royce drill”] penetrated the separate, high-lying Renland ice cap in the Scoresbysund Fiord [...] down to 325 m, world record for this type of drill".[11]
The Renland ice core from East Greenland apparently covers a full glacial cycle from the Holocene into the previous Eemian interglacial. The Renland ice core is 325 m long.[35]
"The δ-profile [...] proved that the Renland ice cap has always been separated from the inland ice. Since all of the δ-leaps revealed by the Camp Century core recurred in the small Renland ice cap, the Renland peninsula cannot have been overrun by ice streams from the inland ice, not even during the glaciation.[11]
GRIP

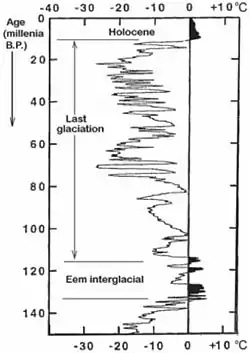
"In 1990 ISTUK started on July 1, and six weeks later it had drilled 710 m. In 1991 we reached 40,000 years old ice at a depth of 2320 m, and July 12, 1992, the drilling was brought to a full stop, when the cutters hit gravel and pebbles at bedrock 3029 m below surface – world record in ice core drilling."[11]
"GRIP (1993) interpreted the climate proxy records from the deepest part of their core as being properly ordered and continuous. They observed large and rapid changes in isotopic temperature with depth and concluded that these features represented rapid changes in climate during marine isotopic Stage 5e, the warmest part of the previous interglacial period. Such a conclusion has extremely important implications for climate because, together with the Dansgaard-Oeschger events, it suggests that rapid cooling events are possible during the current interglacial period."[36]
"Evidence for rapid climate change during Stage 5e rests on the assumption that the deep part of the GRIP ice core is continuous [...] GRIP is located over the present ice divide [...] a recent modeling study has shown that the divide itself has probably migrated [ Anandakrishnan et al., 1993]. Continuity of the deep part of the GRIP core has not yet been definitively demonstrated."[36]
"The most dramatic finding from GRIP was that of the rapid climate changes (Dansgaard-Oeschger events) in the last glacial period. These had been observed in previous cores, but GRIP confirmed their existence, their number, and the extreme rapidity of their onset in unprecedented detail. Such work has stimulated paleoclimatologists in other fields to see how global these events are and to look for causes."[10]
At the right is the "δ-profile along the GRIP ice core. The wildly oscillating record from 1500 to c.2750m depth reflects the turbulent glacial climate."[11]
"Some of the conclusions [from the analysis of the GRIP data are][11]
- The ice core probably reaches more than 150,000 years back in time, i.e. well into the second last glaciation.
- Most of the last glaciation in Greenland (117 kyr to 11.5 kyr B.P.) carries stamp of great and abrupt climate changes, much more violent than in Europe and Antarctica. It was extremely cold about 22 and 70 kyr ago, when Greenland was 25°C colder than now. In post-glacial time, i.e. the last 11.5 kyr the climate has been relatively stable disregarding a short lasting drastic cooling 8250 yrs ago. Roughly, the temperature in Greenland culminated about 9000 yrs ago with mean air temperatures 4°C higher than at present.
- The interglaciation prior to the present one, the Eem period, lasted from 131 to 117 kyr B.P.
- The climate record describes the Eem period as climatically unstable in Greenland with temperatures varying from 5°C warmer to 5°C colder than now, but there is strong evidence that the layer sequence in the deepest 10% of the ice core is disturbed. However, foraminifera records in sediment cores from the western part of the Norwegian Sea show similar trends.
"All of the 24 violent glacial δ shifts appear in all of the long Greenland records. This shows that the mechanism behind them affected at least the entire northwestern part of the North Atlantic Ocean, as would be expected if they were caused by repeated surging of the North American ice sheet".[11]
GISP2


"Ice in GISP2 below 2790 m depth is folded and tilted, and shows evidence of unconformities [ Gow et al., 1993]. The δ18O of O2 in GISP2 above 2790 m matches almost perfectly with the Vostok record [ Sowers et al., 1993]; below that depth, it is far noisier and cannot be aligned with the smoothed Vostok signal [ Bender et al., 1994]. These features all suggest that ice age changes discontinuously in the deepest part of GISP2 as a result of folding, extensive boudinage (squeezing out of layers of ice), and/or intrusion. Bender et al. [1994] concluded that the bottom 200 m of ice at GISP2 may be correctly ordered but discontinuous and extremely condensed, perhaps extending back to several hundred kyr BP. Alternatively, the core may contain a disordered sequence of much younger ice, perhaps largely from about 115-130 kyr BP."[36]
There is evidence the GISP2 cores contain an increasing structural disturbance which casts suspicion on features lasting centuries or more in the bottom 10% of the ice sheet.[37]
At the right is the American GISP2 drill. It towered 15 m up from the top of its gigantic dome through a hole in the ceiling. "The drill was handled from a two storeys scaffold [in the image at the right around the drill], and the operators wore gas masks protecting them against the toxic fumes from the drill fluid, n-butyl acetate, which is harmful to the lungs."[11]
NGRIP
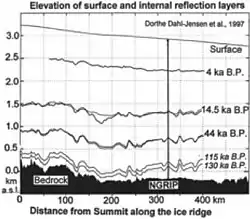
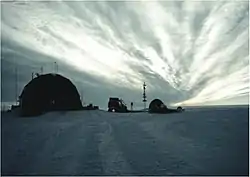
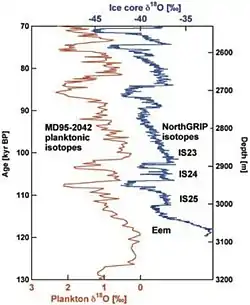
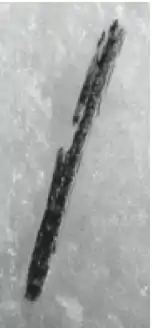
"An apparently favourable location [for a drill site where a deep ice core reveals undisturbed details of the course of climatic events through the entire Emian interglaciation] 320 km NNW of Summit, not far from North Site [...], was found on the basis of a detailed radar sounding [as shown in the image to the right] carried out by the University of Kansas using a special NASA aircraft. The ice movement is very slow and the bedrock flat and horizontal."[11]
"The curves [in the radar profile at the right] are internal reflection layers created by fall-out of acid volcanic debris, i.e. former surfaces and therefore isochrones. Detailed studies suggested undisturbed stratification in the entire ice mass. The Eemian ice seemed to lie 240 m above bedrock."[11]
"The NGRIP drilling site is near the center of Greenland (75.1°N 42.32°W NGRIP drilling site, 2917 m, ice thickness 3085). Drilling began in 1999 and was completed at bedrock in 2003.[38]
In the third figure at the right, "the NGRIP δ record [ref.14.4] through the deepest 454 m is compared with the planktonic δ record through the period 130 to 70 kyrs B.P. dated by radiometric measurements on corals [ref.14.5]. Both of the records are plotted on linear scales (of depth and age, respectively), and the close correlation between them suggests that the ocean time scale may be transferred to the ice core record with some reservations concerning the variability of the accumulation rate at the two drill sites. For example, assuming a constant accumulation rate in the ocean, the relatively narrow minima in the ice core record suggest a lower accumulation rate on the ice sheet in cold periods than under interstadial conditions."[11]
"The correlation is astonishing, because it implies that the dramatic climate changes during the first more than 50 kyrs of the glaciation elapsed nearly in parallel on both sides of the North Atlantic Ocean, presumably controlled by varying sea ice cover. Thus, the Gulf Stream was not just deflected toward North Africa in cold periods, it was rather turned off."[11]
"A comparative interpretation of the two records suggests that the deepest 25 m of the NGRIP core corresponds to the last small maximum in the Eemian part of the ocean record. If so, ice from the onset and the culmination of Eem has melted away".[11]
For the last time, when "the drill came up with 30 cm of light brown refrozen water hanging from the drill head [which included the splinter of wood from a forrest that grew in North Greenland in a distant past shown in the image at the left] bedrock was reached at a depth of 3085.0 m [...] transfer of the radiometrically derived ocean time scale to the NGRIP δ record shows that the mean annual ice layer thickness in the core is fairly constant of the order of 11 mm in the deepest 500 m!".[11]
The NorthGRIP ice core provides an undisturbed record to approx. 123,000 years before present. The results indicate that Holocene climate has been remarkably stable and have confirmed the occurrence of rapid climatic variation during the last ice age.
NEEM
The North Greenland Eemian Ice Drilling (NEEM) site is located at 77°27’N 51°3.6’W, masl. Drilling started in June 2009 and expects to hit bedrock in 2010. The ice at NEEM may be 2545 m thick. As of Sep 1, 2009, the coring has reached a 1757.84 m depth for this season.
Disko Island
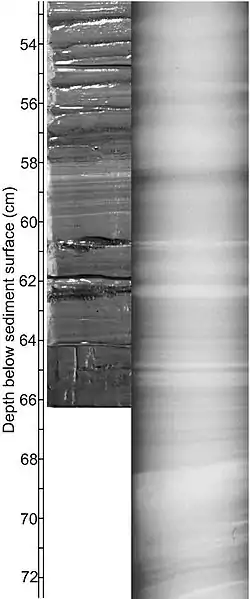
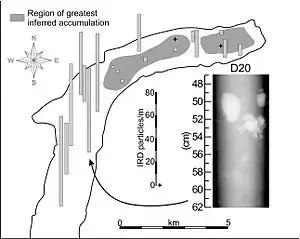
"Beginning in 1995, a large outlet glacier of the Sermersauq Ice Cap on Disko Island [Greenland] surged 10.5 km downvalley to within 10 km of the head of the fjord, Kuannersuit Sulluat, reaching its maximum extent in summer 1999 before beginning to retreat. Sediment discharge to the fjord increased from 13 x 103 t day-1 in 1997 to 38 x 103 t day-1 in 1999. CTD results, sediment traps and cores from the 2000 melt season document the impact of the surge on the glacimarine environment of the fjord."[39]
"Short gravity cores were taken and CTD profiles were recorded at stations throughout Kuannersuit Sulluat [...]. Positions located by GPS are accurate to ±10 m or less. The stream flowing over the sandur to the head of the fjord was gauged and integrated suspended sediment samples were recovered from primary channels."[39]
"The cores were photographed, X-rayed and logged. X-radiographs provided measures of the number and size of gravel particles interpreted as ice-rafted debris (IRD) and the grey-scale (GS) of the scanned images was plotted as a measure of the properties of the sand and silt."[39]
"The twelve layers in core D4 [imaged at the right] suggest a mean period of about 20 days for these events based on the accumulation rates in the traps [...]. In general, these layers have both higher MS and X-radiographs have lighter toned GS, the former related to lower water content and the latter also related to greater absorption of X-rays by the larger rock and mineral fragments."[39]
There "are notable differences in the surge-generated sediments. The proximal sediments [such as in core D4 at the right] are more clearly laminated and layered in visual examination of the cores and as seen in the X-radiographs [compared to distal sediments as imaged on the left for core D20]. These consist both of the subtle differences in the fine-grained sediments on a millimetre scale, and of the sand layers up to 8 cm thick representing more energetic processes (Ó Cofaigh and Dowdeswell, 2001). Both are a response to greater sediment input to the fjord."[39]
Antarctica
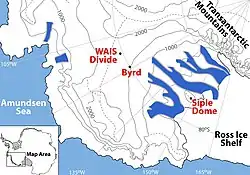
"Among numerous other findings, new insights using markers of biological material have proved particularly exciting. Methane has been found to change in time with many rapid climate changes. Spikes of ammonium and organic acids have been found to be markers for biomass burning, while background concentrations of these species indicate the advances of vegetation in North America."[10]
Byrd Station

Marie Byrd Land formerly hosted the Operation Deep Freeze base Byrd Station (NBY), beginning in 1957, in the hinterland of Bakutis Coast. Byrd Station was the only major base in the interior of West Antarctica. In 1968, the first ice core to fully penetrate the Antarctic Ice Sheet was drilled here.
The Byrd 1968 core was 2164 m to bedrock and exhibited the post-glacial climatic optimum correlateably well with the Camp Century 1963 core from Greenland.[11]
The drill tube is at the top of the image.
Plateau Station
Plateau Station is an inactive American research and Queen Maud Land traverse support base on the central Antarctic Plateau. The base was in continuous use until January 29, 1969. Ice core samples were made, but with mixed success.
Dolleman Island
The British Antarctic Survey (BAS) has used Dolleman Island as ice core drilling site in 1976, 1986 and 1993.
Mizuho Station
- Narita, H., Y. Fujii, Y. Nakayama, K. Kawada and A. Takahashi, 1994, Thermal ice core drilling to 700 m depth at Mizuho Station, East Antarctica, Memoirs of National Institute of Polar Research, Special Issue No. 49, Pages 172-183.
Berkner Island
In the 1994/1995 field season the British Antarctic Survey, Alfred Wegener Institute for Polar and Marine Research and Alfred Wegener Institute and the Forschungsstelle für Physikalische Glaziologie of the University of Münster cooperated in a project drilling ice cores on the North and South Domes of Berkner Island.
Dome F
Two deep ice cores were drilled near the Dome F summit (77° 19' S 39° 42' E, altitude 3,810 m). The first drilling started in August 1995, reached a depth of 2503 m in December 1996 and covers a period back to 320,000 years. The second drilling started in 2003, was carried out during four subsequent austral summers from 2003/2004 until 2006/2007, and by then a depth of 3,035.22 m was reached. This core greatly extends the climatic record of the first core, and, according to a first, preliminary dating, it reaches back until 720,000 years.
Cape Roberts
Between 1997 and 1999 the international Cape Roberts Project (CRP) has recovered up to 1000 m long drill cores in the Ross Sea, Antarctica to reconstruct the glaciation history of Antarctica.
Dronning Maud Land
- Drucker, C., F. Wilhelms, H. Oerter, A. Frenzel, H. Gernandt and H. Miller, 2002, Design, transport, construction, and operation of the summer base Kohnen for ice-core drilling in Dronning Maud Land, Antarctica, Memoirs of National Institute of Polar Research, Special Issue No. 56, Pages 302-312.
Vostok
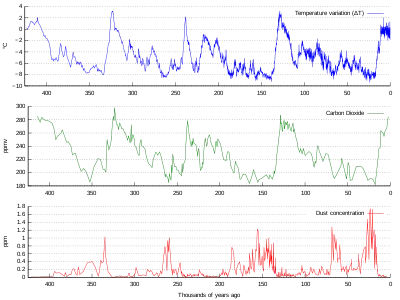
As of 2003, the longest core drilled was at [Vostok, Antarctica] Vostok station. It reached back 420,000 years and revealed 4 past glacial cycles. Drilling stopped just above Lake Vostok. The Vostok core was not drilled at a summit, hence ice from deeper down has flowed from upslope; this slightly complicates dating and interpretation. Vostok core data are available.[40]
For the list of ice cores visit IceReader web site
EPICA/Dome C
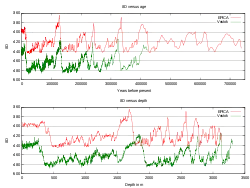
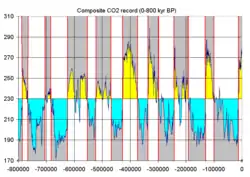
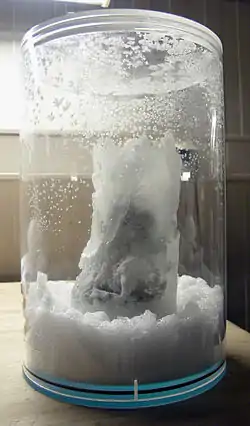
The European Project for Ice Coring in Antarctica (EPICA) first drilled a core near Dome C at 75° S 123° E (560 km from Vostok) at an altitude of 3,233 m. The ice thickness is 3,309 +/-22 m and the core was drilled to 3,190 m. It is the longest ice core on record, where ice has been sampled to an age of 800 kyr BP (Before Present).[41] Present-day annual average air temperature is -54.5 °C and snow accumulation 25 mm/y. Information about the core was first published in Nature on June 10, 2004. The core revealed 8 previous glacial cycles.
At the left is the end of the EDML ice core from a depth 2775 m. It consists of meltwater which has formed at the base of the ice sheet. The core was drilled 2002-2004 through the Antarctic ice sheet in the area of Dronning Maud Land by the project EPICA (European Project for Ice Coring in Antarctica).
The "3,270 metre-long ice core completed in 2004 from Dome C in Antarctica [...] although not the longest core ever recovered in Antarctica (that record goes to a 3,650 metre core from Vostok), the EPICA core is the oldest, stretching back 800,000 years."[42]
"It's not just a case of drilling deeper, because an ice sheet is at its coldest at the surface and its warmest at the bed. The ice sits on the Earth's mantle like a blanket, and the thicker the blanket, the harder it is for the heat to escape. If the ice sheet is too thick, the oldest ice at the bottom of the core will have melted."[16]
"Deciding where to drill depends on the question you want to answer, but generally, ice core scientists tend to choose somewhere that's a dome, where the bottom of the ice sheet is relatively flat and where there is little ice flow, because as you drill deeper, you don't want to be drilling into ice that has flowed from somewhere else. We need to find somewhere where the ice isn't much thicker than at Dome C, but where it's colder at the surface. That will mean that it's also colder at the base, so the oldest ice will have stayed frozen. We also want a place with a low accumulation rate so that the ice ages faster as you get deeper."[16]
"Given these criteria, the best bet in the hunt for a 1.4 million year ice core looks like Dome A, more than 4,000 metres above sea level and over 1,000 kilometres from the nearest research station. Before drilling begins, aircraft will survey the area to discover the shape of the bed and how much ice lies above it, and select the best possible site. Even then, drilling will be tough."[42]
"The challenge of working at Dome A will be the high altitude - it's at more than 4,000 metres and because the Earth's atmosphere is thinner in the polar regions, that's like working at between 5,000 and 6,000 metres above sea level. Even working at Dome C - which is at 3,300 metres - had me gasping until I acclimatised."[16]
Although the major events recorded in the Vostok, EPICA, NGRIP, and [Greenland ice core project] GRIP during the last glacial period are present in all four cores, some variation with depth (both shallower and deeper) occurs between the Antarctic and Greenland cores.
West Antarctic Ice Sheet Divide
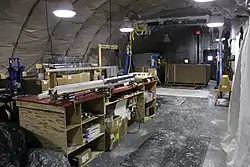
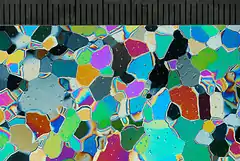
The West Antarctic Ice Sheet Divide (WAIS Divide) Ice Core Drilling Project began drilling over the 2005 and 2006 seasons, drilling ice cores up to the depth of 300 m for the purposes of gas collection, other chemical applications, and to test the site for use with the Deep Ice Sheet Coring (DISC) Drill. Sampling with the DISC Drill will begin over the 2007 season and researchers and scientists expect that these new ice cores will provide data to establish a greenhouse gas record back over 40,000 years.
"The different colors [in the thin section at the left] are due to the different orientations of the crystals. The small circles are entrapped atmospheric air bubbles. These samples of ancient air provide scientists and policy-makers with direct evidence of past atmospheric composition."[43]
Kohnen Station
They subsequently drilled a core at Kohnen Station in 2006.[44]
International Trans-Antarctic Scientific Expedition (ITASE)
The International Trans-Antarctic Scientific Expedition (ITASE) was created in 1990 with the purpose of studying climate change through research conducted in Antarctica. A 1990 meeting held in Grenoble, France, served as a site of discussion regarding efforts to study the surface and subsurface record of Antarctica’s ice cores.
TAlos Dome Ice CorE Project
TAlos Dome Ice CorE Project is a new 1620 m deep ice core drilled at Talos Dome that provides a paleoclimate record covering at least the last 250,000 years. The TALDICE coring site (159°11'E 72°49'S; 2315 m a.s.l.; annual mean temperature -41°C) is located near the dome summit and is characterised by an annual snow accumulation rate of 80 mm water equivalent.[45]
Non-polar cores
The non-polar ice caps, such as found on mountain tops, were traditionally ignored as serious places to drill ice cores because it was generally believed the ice would not be more than a few thousand years old, however since the 1970s ice has been found that is older, with clear chronological dating and climate signals going as far back as the beginning of the most recent ice age. Although polar cores have the clearest and longest chronological record, four-times or more as long, ice cores from tropical regions offer data and insights not available from polar cores and have been very influential in advancing understanding of the planets climate history and mechanisms.
Mountain ice cores have been retrieved in the Andes in South America, Mount Kilimanjaro in Africa, Tibet, various locations in the Himalayas, Alaska, Russia and elsewhere. Mountain ice cores are logistically very difficult to obtain. The drilling equipment must be carried by hand, organized as a mountaineering expedition with multiple stage camps, to altitudes upwards of 20,000 feet (helicopters are not safe), and the multi-ton ice cores must then be transported back down the mountain, all requiring mountaineering skills and equipment and logistics and working at low oxygen in extreme environments in remote third world countries. Scientists may stay at high altitude on the ice caps for up 20 to 50 days setting altitude endurance records that even professional climbers do not obtain.
Mount Kilimanjaro
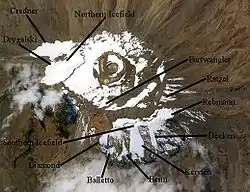
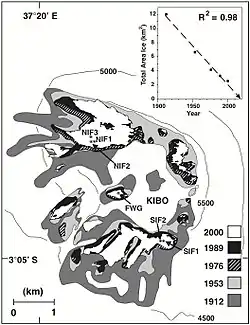

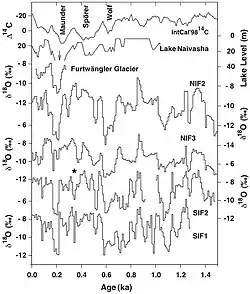
"Aerial photographs taken on 16 February 2000 allowed production of a recent detailed map of ice cover extent on the summit plateau [diagram at the left]."[46]
"Six ice cores from Kilimanjaro provide an ~11.7-thousand-year record of Holocene climate and environmental variability for eastern equatorial Africa, including three periods of abrupt climate change: ~8.3, ~5.2, and ~4 thousand years ago (ka, [b2k]). The latter is coincident with the “First Dark Age,” the period of the greatest historically recorded drought in tropical Africa. Variable deposition of F– and Na+ during the African Humid Period suggests rapidly fluctuating lake levels between ~11.7 and 4 ka [b2k]."[46]
"The three longest cores (NIF1, NIF2, and NIF3) were drilled to depths of 50.9, 50.8, and 49.0 m, respectively, from the Northern Ice Field (NIF), the largest of the ice bodies. Two shorter cores (SIF1 and SIF2) were drilled to bedrock on the Southern Ice Field (SIF) to depths of 18.5 and 22.3 m, respectively, and a 9.5-m core was drilled to bedrock on the small, thin Furtwa ̈ngler Glacier (FWG) within the crater. Temperatures were measured in each bore-hole; in the NIF, they ranged from –1.2°C at 10 m depth to –0.4°C at bedrock, and in the SIF, they were near 0°C. No evidence of water was observed in the boreholes on the NIF or SIF, but the FWG was water-saturated throughout."[46]
"The chemical and physical analyses, coupled with visible stratigraphy for near-surface layers of the NIF2 core, are shown in [the image at the lower right, A]. Melt features similar to those in the top meter did not occur elsewhere in the NIF or SIF cores. The ongoing down-wasting of the ice fields had not yet removed the ice deposited in the early 1950s, because snow was recovered that contained elevated concentrations of 36Cl from the 1952 Ivy thermonuclear bomb test on Eniwetok Atoll (6). The 1952 time horizon, used for time control in other low-latitude ice cores [the image at the lower right, B], provided a logical origin for development of a depth-age model for the suite of Kilimanjaro cores."[46]
"Water levels in Lake Naivasha, Kenya, [lower diagram at the left,] show higher stands during all three recent solar minima (Maunder, Spörer, and Wolf) with a ~100-year (1670 to 1780 A.D.) period of overflow coincident with the Maunder Minimum. The earliest of the three high stands of Lake Naivasha is 14C-dated between 1290 and 1370 A.D., and the close association between the water balance in East Africa and solar variability (10) argues for a relationship between the NIF δ 18O minima and the solar minima [lower diagram at the left]."[46]
The African Humid Period began ~11,000 b2k until 4,000 b2k, "when warmer and wetter conditions prevailed (14, 15) in response to the precession-driven increase in solar radiation (16). During this interval, lakes in the region rose as much as 100 m above present levels (14, 17), and in sub-Saharan Africa lake expansion was massive, with Lake Chad expanding 25-fold from ~17,000 km2 to cover an area between ~330,000 and 438,000 km2, comparable to that of the Caspian Sea today (14, 18, 19). A paleolake filled the Magadi Natron basin on the border between Tanzania and Kenya to a depth 50 m above the present level and had an area of ~1600 km2 in the early Holocene (20)."[46]
"The Kilimanjaro record documents three abrupt climate changes in this region: at ~8.3, ~5.2, and ~4 ka."[46]
These three periods correlate with similar events in the Greenland GRIP and GISP2 cores.[46]
Because glaciers are retreating rapidly worldwide, some important glaciers are now no longer scientifically viable for taking cores, and many more glacier sites will continue to be lost, the "Snows of Mount Kilimanjaro" (Hemingway) for example could be gone by 2015.[47]
Guliya
The ice core drilled in Guliya ice cap in western China in the 1990s reaches back to 760,000 b2k; farther back than any other core at the time, though the EPICA core in Antarctica equalled that extreme in 2003.[48]
Lake Vida
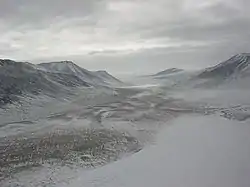
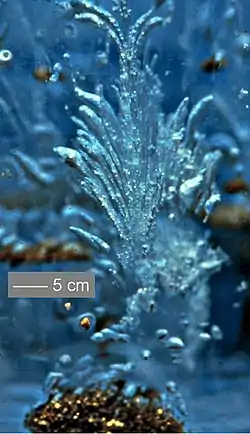
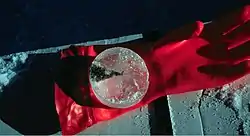
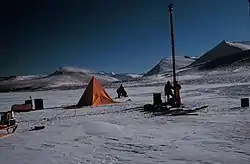
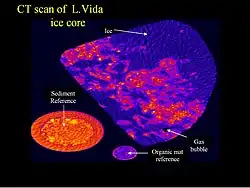
"Antarctic Lake Vida may represent a previously unknown ecosystem, a frigid, "ice-sealed," lake that contains the thickest non-glacial lake ice cover on Earth and water seven times saltier than seawater."[49]
Using "ground-penetrating radar, ice core analyses, and long-term temperature data, the researchers now show that Vida has a thick light-blocking ice cover, a vast amount of ancient organic material and sediment, and a cold, super-salty, liquid zone underlying the ice -- an environment that remains liquid at temperatures under -10°C, well below the freezing point of pure water."[49]
"The researchers extracted two ice cores from Lake Vida [using the rig at the lower left] in early Antarctic spring (October) 1996. With an electromechanical drill, team members spent two weeks at temperatures below -35°C drilling a 10-cm-diameter core through 16-m of ice cover."[49]
The second image at the right shows a small segment of one of the ice cores from Lake Vida with a layer of sediment. A computed tomography (CT) image third lowest at the right of a section of an ice core from Lake Vida shows the organic (microbe 2,800 year old halophile microbes (primarily filamentous cyanobacteria) containing) material surrounding sediment in an icy matrix.
"The sediment within the ice made coring extremely difficult and required frequent bit changes and a complete motor replacement at one stage."[50]
"It was some very cold drilling. We were there for two weeks at temperatures approaching -40°C ... camping. The drillers had a hard time getting through the sediment layers. They were used to drilling clean ice up on the polar plateau; the dirt in the ice tended to dull the cutting bits."[51]
"The ice covers of these lakes represent an oasis for life in an environment previously thought to be inhospitable. These life forms may possess novel ice-active substances such as antifreezes and ice nucleation inhibitors that allow the organisms to survive the freeze-thaw cycles and come back to life when exposed to liquid water."[50]
"Importantly, the cold temperatures preserve DNA extremely well making them perfect 'ice museums' for the study of ancient DNA."[50]
"Lake Vida, more than 5 km long, is one of the largest in the cold Antarctic desert region known as the McMurdo Dry Valleys. The area receives less than 10 cm of snow per year and the average annual temperature hovers around -30°C."[49]
"Lake Vida provides insight into a novel terrestrial ecosystem. What happened at Lake Vida may have been the fate of other Antarctic lakes, during even colder times, and more tropical aquatic ecosystems during extreme global glaciations of the past, such as the 'snowball Earth' 550 Million years ago."[51]
"Lake Vida in the [foreground of the image at the top right] apparently has no ice on it, but in fact this is summer meltwater that has flowed onto the surface of the thick permanent Lake Vida ice cover. In the image is about 1 m of muddy water above 19 m of ice, all sitting on top of a hypersaline brine."[49]
"The bubbles [such as in the image at the top left] tell us that there was liquid water present above the sediment at one time and that the water froze from the top down."[49]
Upper Fremont Glacier

Ice core samples were taken from Upper Fremont Glacier in 1990-1991. These ice cores were analyzed for climatic changes as well as alterations of atmospheric chemicals. In 1998 an unbroken ice core sample of 164 m was taken from the glacier and subsequent analysis of the ice showed an abrupt change in the oxygen isotope ratio oxygen-18 to oxygen-16 in conjunction with the end of the Little Ice Age, a period of cooler global temperatures between the years 1550 and 1850. A linkage was established with a similar ice core study on the Quelccaya Ice Cap in Peru. This demonstrated the same changes in the oxygen isotope ratio during the same period.
An ice core has been analyzed, as shown on the right, for mercury over the last 270 years.
Nevado Sajama
Ice cores from Sajama in Bolivia span ~25 ka and help present a high resolution temporal picture of the Late Glacial Stage and the Holocene climatic optimum.[52]
Huascarán
Ice cores from Huascarán in Peru like those from Sajama span ~25 ka and help present a high resolution temporal picture of the Late Glacial Stage and the Holocene climatic optimum.[52]
Quelccaya Ice Cap
Although the ice cores from Quelccaya ice cap only go back ~2 ka,[52] others may go back ~5.2 ka. The Quelccaya ice cores correlate with those from the Upper Fremont Glacier.
East Rongbuk Glacier
A shallow ice core drilled from the East Rongbuk glacier showed a dramatic increasing trend of black carbon concentrations in the ice stratigraphy since the 1990s.[53]
History
"The first to winter on the [Greenland] inland ice was [...] J.P. Koch and the German meteorologist Alfred Wegener. In 1912 they built a hut on the ice in Northeast Greenland [...] Wegener studied the weather conditions throughout the winter, and inside the hut they drilled to a depth of 25 metres by an auger not unlike an oversized corkscrew. They measured the temperature at various depths and its variation throughout the winter."[11]
Technology
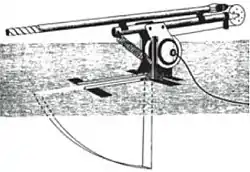

"The “Rolls Royce drill ” is based on the same principles as the SIPRE auger. It weighs c. 250 kg, incl. winch, “tower”, 200m cable, electronic control, foundation and generator. The drill can be tilted from horizontal to vertical position [as in the photograph at the left], the lower end going into a 11⁄2 m deep trench in the snow. A 100 m long ice core can be drilled and packed in 16 hours by three persons. In the drawing [at the right], the inner core barrel has been pulled partly out of the outer one."[11]
"“Shallow drilling” means ice core drilling to a depth of up to 100 m, that is through the youngest 200-500 annual layers of the inland ice."[11]
See also
References
- 1 2 3 M. Gerasimoff (2003). Drilling Fluid Observations and Recommendations for U.S. Polar Program, WAISCORES Drilling Project. University of Wisconsin-Madison: Ice Coring and Drilling Services. Retrieved 2014-10-07.
- ↑ C. SIMON L. OMMANNEY. HISTORY OF GLACIER INVESTIGATIONS IN CANADA (PDF). Retrieved October 14, 2005.
- ↑ Amy Ng and Clair Patterson (1981). "Natural concentrations of lead in ancient Arctic and Antarctic ice". Geochimica et Cosmochimica Acta 45 (11): 2109–21. doi:10.1016/0016-7037(81)90064-8.
- ↑ Brent Christner. Glacial ice cores: a model system for developing extraterrestrial decontamination protocols. Retrieved May 23, 2005.
- ↑ Physical and structural properties of the GISP2 ice core. http://www.gisp2.sr.unh.edu/GISP2/Contri_Series/full/09.html#8. Retrieved October 14, 2005.
- ↑ Physical Properties Research on the GISP2 Ice Core. Retrieved October 14, 2005.
- ↑ Svensson, A., S. W. Nielsen, S. Kipfstuhl, S. J. Johnsen, J. P. Steffensen, M. Bigler, U. Ruth, and R. Röthlisberger (2005). "Visual stratigraphy of the North Greenland Ice Core Project (NorthGRIP) ice core during the last glacial period". J. Geophys. Res. 110 (D02108): D02108. doi:10.1029/2004JD005134.
- ↑ A.J. Gow and D.A. Meese. The Physical and Structural Properties of the Siple Dome Ice Cores, In: WAISCORES. Retrieved October 14, 2005.
- ↑ Jane Beitler (2014). Cryosphere Glossary. National Snow and Ice Data Center. Retrieved 2014-09-17.
- 1 2 3 B. Stauffer (1992). The GRIP Ice Coring Effort. Washington, DC USA: NOAA. Retrieved 2014-08-24.
- 1 2 3 4 5 6 7 8 9 10 11 12 13 14 15 16 17 18 19 20 21 22 23 24 25 26 27 28 29 30 31 32 33 34 35 36 37 38 39 Willi Dansgaard (2005). The Department of Geophysics of The Niels Bohr Institute for Astronomy Physics and Geophysics at The University of Copenhagen Denmark (ed.). Frozen Annals Greenland Ice Cap Research. Copenhagen, Denmark: Niels Bohr Institute. p. 123. ISBN 87-990078-0-0. Retrieved 2014-10-05.
- 1 2 3 Carol Kendall (January 2004). Resources on Isotopes. Menlo Park, California USA: United States Geological Survey. Retrieved 2014-10-05.
- ↑ Atmospheric Research (October 2014). BUBBLES IN ICE. CSIRO. Retrieved 2014-10-08.
- ↑ NOAA Paleoclimatology Program — Vostok Ice Core Timescales. Retrieved October 14, 2005.
- ↑ Polar Paleo-Climate Interests. Retrieved October 14, 2005.
- 1 2 3 4 5 Robert Mulvaney (20 October 2010). The ice man cometh - ice cores reveal past climates. British Antarctic Survey. Retrieved 2014-10-09.
- ↑ Michael Bender, Todd Sowers, and Edward Brook (August 1997). "Gases in ice cores". Proceedings of the National Academy of Sciences of the United States of America 94 (16): 8343–9. doi:10.1073/pnas.94.16.8343. PMID 11607743. PMC 33751. //www.ncbi.nlm.nih.gov/pmc/articles/PMC33751/.
- ↑ TRENDS: ATMOSPHERIC CARBON DIOXIDE. Retrieved October 14, 2005.
- ↑ Hubertus Fischer, Martin Wahlen, Jesse Smith, Derek Mastroianni, Bruce Deck (1999-03-12). "Ice Core Records of Atmospheric CO2 Around the Last Three Glacial Terminations". Science (Science) 283 (5408): 1712–4. doi:10.1126/science.283.5408.1712. PMID 10073931. http://www.sciencemag.org/cgi/content/abstract/283/5408/1712?maxtoshow=&HITS=10&hits=10&RESULTFORMAT=&fulltext=Fischer%2C+H.%2C+M.+Wahlen%2C+J.+Smith%2C+D.+Mastroiani+and+B.+Deck%2C+1999%3A+Ice+core+records+of+atmospheric+CO2+around+the+last+three+glacial+terminations.+Science%2C+283%2C+1712-1714.&searchid=1&FIRSTINDEX=0&resourcetype=HWCIT. Retrieved 2010-06-20.
- ↑ Purdue study rethinks atmospheric chemistry from ground up. Retrieved October 14, 2005.
- ↑ Summit_ACS.html. Retrieved October 14, 2005.
- ↑ Jim White and Eric Steig. Siple Dome Highlights: Stable isotopes, In: WAISCORES. Retrieved October 14, 2005.
- 1 2 3 4 Paleo (20 August 2008). Penny Ice Cap. Bethsda, Maryland USA: NOAA. Retrieved 2014-11-14.
- 1 2 3 CC Langway Jr. (January 2008). The History of Early Polar Ice Cores (PDF).
- ↑ Kurt Wegener (September 1955). "Die Temperatur in Grönlandischen Inlandeis". Geofisica Pura e Applicata 32 (1): 102-6. doi:10.1007/BF01993599. http://link.springer.com/article/10.1007/BF01993599.
- ↑ Dansgaard W, Johnsen SJ, Møller J, Langway Jr CC (October 1969). "One Thousand Centuries of Climatic Record from Camp Century on the Greenland Ice Sheet". Science 166 (3903): 377-80. doi:10.1126/science.166.3903.377.
- ↑ Oeschger H, Beer J, Andree M (August 1987). "10Be and 14C in the Earth system". Phil Trans R Soc Lond A. 323 (1569): 45-56.
- ↑ Rose LE (Winter). "The Greenland Ice Cores". Kronos 12 (1): 55-68.
- ↑ Crete Ice Core.
- ↑ NOAA Paleoclimatology World Data Centers Dye 3 Ice Core.
- ↑ Shoji, Langway Jr CC (Aug). Nature.: 548.
- 1 2 3 Dansgaard W, Clausen HB, Gundestrup NS, Hammer CU, Johnsen SJ, Kristinsdottir PM, Reeh (Dec 1982). "A new greenland deep ice core". Science. 218 (4579): 1273–77 [1274]. doi:10.1126/science.218.4579.1273. PMID 17770148.
- ↑ Rose LE (Winter 1987). "Some preliminary remarks about ice cores". Kronos. 12 (1): 43–54.
- ↑ Herron, Herron, Langway (Oct). Nature.: 389.
- ↑ Hansson M, Holmén K (November 2001). Geophy Res Lett. 28 (22): 4239-42. doi:10.1029/2000GL012317.
- 1 2 3 GISP2 and GRIP Records Prior to 110 kyr BP. Archived from the original on September 9, 2005. Retrieved October 14, 2005.
- ↑ Gow, A. J., D. A. Meese, R. B. Alley, J. J. Fitzpatrick, S. Anandakrishnan, G. A. Woods, and B. C. Elder (1997). "Physical and structural properties of the Greenland Ice Sheet Project 2 ice core: A review". J. Geophys. Res. 102 (C12): 26559–76. doi:10.1029/97JC00165.{
- ↑ David Whitehouse (14 October 2005). "Breaking through Greenland's ice cap". BBC.
- 1 2 3 4 5 Robert Gilbert, Niels Nielsen, Henrik Möller, Joseph R. Desloges, and Morten Rasch (2002). "Glacimarine sedimentation in Kangerdluk (Disko Fjord), West Greenland, in response to a surging glacier". Marine Geology 191: 1-18. http://geog.queensu.ca/gilbert/surge%20paper.PDF. Retrieved 2014-09-24.
- ↑ NOAA Paleoclimatology Program — Vostok Ice Core. Retrieved October 14, 2005.
- ↑ Deep ice tells long climate story. September 4, 2006. Retrieved May 4, 2010.
- 1 2 Becky Allen (20 October 2010). The ice man cometh - ice cores reveal past climates. British Antarctic Survey. Retrieved 2014-10-09.
- ↑ John Fegyveresi (2011). WAIS Divide Ice Core: Horizontal. flickr. Retrieved 2014-08-25.
- ↑ Mark Peplow (2006). "Ice core shows its age". News@nature. doi:10.1038/news060123-3.
- ↑ http://www.taldice.org
- 1 2 3 4 5 6 7 8 Lonnie G. Thompson, Ellen Mosley-Thompson, Mary E. Davis, Keith A. Henderson, Henry H. Brecher, Victor S. Zagorodnov, Tracy A. Mashiotta, Ping-Nan Lin, Vladimir N. Mikhalenko, Douglas R. Hardy, Jürg Beer (18 October 2002). "Kilimanjaro Ice Core Records: Evidence of Holocene Climate Change in Tropical Africa". Science 298 (5593): 589-93. doi:10.1126/science.1073198. ftp://ftp.soest.hawaii.edu/engels/Stanley/Textbook_update/Science_298/Thompson-02.pdf. Retrieved 2014-10-04.
- ↑ Deciphering the ice. CNN. 12 September 2001. Retrieved 8 July 2010.
- ↑ Mark Bowen (2005). Thin Ice. Henry Holt Company. ISBN 0-8050-6443-5.
- 1 2 3 4 5 6 Josh Chamot and Polly Penhale (16 December 2002). Researchers Uncover Extreme Lake -- and 3000-Year-Old Microbes -- in Mars-Like Antarctic Environment. Arlington, Virginia USA: U. S. National Science Foundation. Retrieved 2014-10-10.
- 1 2 3 John Priscu (16 December 2002). Researchers Uncover Extreme Lake -- and 3000-Year-Old Microbes -- in Mars-Like Antarctic Environment. Arlington, Virginia USA: U. S. National Science Foundation. Retrieved 2014-10-10.
- 1 2 Peter Doran (16 December 2002). Researchers Uncover Extreme Lake -- and 3000-Year-Old Microbes -- in Mars-Like Antarctic Environment. Arlington, Virginia USA: U. S. National Science Foundation. Retrieved 2014-10-10.
- 1 2 3 Thompson LG, Mosley-Thompson EM, Henderson KA (2000). "Ice-core palaeoclimate records in tropical South America since the Last Glacial Maximum". J Quaternary Sci. 15 (4): 377-94. doi:10.1002/1099-1417(200005)15:4<377::AID-JQS542>3.0.CO;2-L.
- ↑ Ming J, Cachier H, Xiao C, "et al." (2008). ACP 8 (5): 1343-52.
Further reading
- http://www.tonderai.co.uk/earth/ice_cores.php "The Chemistry of Ice Cores" literature review
- Barnola J, Pimienta P, Raynaud D, Korotkevich Y (1991). "CO2-Climate relationship as deduced from the Vostok ice core – a reexamination based on new measurements and on a reevaluation of the air dating". Tellus Series B-Chemical and Physical Meteorology 43 (2): 83–90. doi:10.1034/j.1600-0889.1991.t01-1-00002.x.
- Battle M, Bender M, Sowers T, et al (1996). "Atmospheric gas concentrations over the past century measured in air from firn at the South Pole". Nature 383 (6597): 231–5. doi:10.1038/383231a0.
- Friedli H, Lotscher H, Oeschger H, et al (1986). "Ice core record of the C13/C12 ratio of atmospheric CO2 in the past two centuries". Nature 324 (6094): 237–8. doi:10.1038/324237a0.
- Andersen KK, Azuma N, Barnola JM, et al. (September 2004). "High-resolution record of Northern Hemisphere climate extending into the last interglacial period" (PDF). Nature 431 (7005): 147–51. doi:10.1038/nature02805. PMID 15356621. http://homepages.ulb.ac.be/~desamyn/NATURE02805_published-version_09-09-04.pdf.
- Alley RB (February 2000). "Ice-core evidence of abrupt climate changes". Proc. Natl. Acad. Sci. U.S.A. 97 (4): 1331–4. doi:10.1073/pnas.97.4.1331. PMID 10677460. PMC 34297. http://www.pnas.org/cgi/pmidlookup?view=long&pmid=10677460.
- Ice Cores: A Window into Climate History August 2010
- BBC: "Core reveals carbon dioxide levels are highest for 800,000 years" September 2006
- "Ice cores unlock climate secrets" BBC June 2003
- "Frozen time" June 2004
- "New Ice Core Record Will Help Understanding of Ice Ages, Global Warming" June 2004
- "Oldest ever ice core promises climate revelations" New Scientist September 2003]
External links
- Ice Core Gateway
- National Ice Core Laboratory - Facility for storing, curating, and studying ice cores recovered from the polar regions.
- Ice-core evidence of rapid climate shift during the termination of the Little Ice Age - Upper Fremont Glacier study
- Byrd Polar Research Center - Ice Core Paleoclimatology Research Group
- National Ice Core Laboratory - Science Management Office
- West Antarctic Ice Sheet Divide Ice Core Project
- PNAS Collection of Articles on the Rapid Climate Change
- Map of some worldwide ice core drilling locations
- Map of some ice core drilling locations in Antarctica
- U.S. Ice Drilling Program Library