
A laboratory is a specialized activity where a student, teacher, or researcher can have hands-on, or as close to hands-on as possible, experience actively analyzing an entity, source, or object of interest.
Usually, expensive equipment, instruments, and/or machinery are available for taking the entity apart to see and accurately record how it works, what it's made of, and where it came from. This may involve simple experiments to test reality, collect data, and try to make some sense out of it.
Expensive equipment can be replaced or substituted for with more readily available tools.
![]() Evaluation
|
Notations
You are free to create your own notation or use that already presented. A method to statistically assess your locator is also needed.
Laboratory control group
A laboratory control group of some large number of laboratory test subjects or results may be used to define normal limits for the presence of an effect.
Laboratory
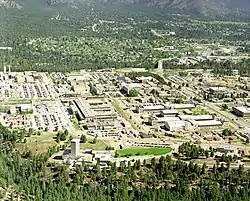
Def. "a room, building or institution equipped for scientific research, experimentation or analysis"[1] is called a laboratory.
Instructions
This laboratory is an activity for you to explore the universe for, to create a method for, or to examine. While it is part of the astronomy course principles of radiation astronomy, it is also independent.
Some suggested entities to consider are
- available classification,
- electromagnetic radiation,
- particle or meteor radiation,
- plasma, gaseous, liquid, or solid propagation,
- classical physics quantities, or
- geometry.
More importantly, there are your entities.
You may choose to define your entities or use those already available.
Usually, research follows someone else's ideas of how to do something. But, in this laboratory you can create these too.
This is an astronomy laboratory, but you may create what a laboratory, or an astronomy is.
Yes, this laboratory is structured.
I will provide an example. The rest is up to you.
Questions, if any, are best placed on the discussion page.
To include your participation in each of these laboratories create a subpage of your user page once you register at wikiversity and use this subpage, for example, [[User:your online name|your online name/laboratory effort.
Enjoy learning by doing!
Samplings
Once you've decided on an entity, source, or object, compose a method, way, or procedure to explore it.
One way is to perceive (see, feel, hear, taste, or touch, for example) if there are more than one of them.
Ask some questions about it.
Does it appear to have a spatial extent?
Is there any change over time?
Can it be profiled with a kind of spectrum for example, by emitted radiation? Sample by plotting two or more apparent variables against each other, like intensity versus wavelength.
Is there some location, time, intensity, where there isn't one?
Verifications
To verify that your sampling has explored something, you may need a control group. Perhaps where, when, or without your entity, source, or object may serve.
Another verifier is reproducibility. Can you replicate something about your entity in your laboratory more than 3 times. Five times is usually a beginning number to provide statistics (data) about it.
For an apparent one time or perception event, document or record as much information coincident as possible. Was there a butterfly nearby?
Has anyone else perceived the entity and recorded something about it?
Laboratory reports
Below is an outline for sections of a report, paper, manuscript, log book entry, or lab book entry. You may create your own, of course.
Title
by line
Abstract
Introduction
Experiment
Results
Discussion
Conclusion
Laboratory evaluations
To assess your example, including your justification, analysis and discussion, I will provide such an assessment of my example for comparison and consideration.
Evaluation
Analytical astronomy laboratory
Astrognosy laboratory
Cosmogony laboratory
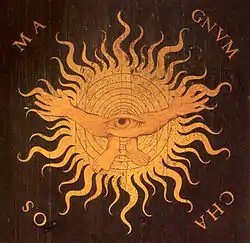
This laboratory is an activity for you to create a universe. While it is part of the astronomy course principles of radiation astronomy, it is also independent.
Some suggested primordial entities to consider are electromagnetic radiation, neutrinos, mass, time, Euclidean space, Non-Euclidean space, and spacetime.
More importantly, there are your primordial entities. And, yes, you can create a universe from a peanut butter and jelly sandwich if you wish to.
You may choose to define your primordial entities or not.
Usually, research follows someone else's ideas of how to do something. But, in this laboratory you can create these too.
Okay, this is an astronomy cosmogony laboratory, but you may create what an astronomy, a cosmogony, or a laboratory is.
Yes, this laboratory is structured. And, you are providing it. Or, not, an unstructured universe is okay too.
I will provide one or a few examples of cosmogonies. The rest is up to you.
Questions, if any, are best placed on the discussion page.
Cosmogony example
Electromagnetism exists. It is neither finite not infinite but uncountable.
As a characteristic, magnetic field and electric field are at 90° to each other.
Constructive interference results in a tangle. Destructive interference results in a tachyonic tangle.
The appearance of Euclidean dimensions occurs from tangles within the electromagnetism.
The appearance of motion occurs as tangles increase, decrease, disentangle or self-propagate through repulsion or attraction.
Evaluation of cosmogony example
Is this cosmogony for the universe? Yes.
What came before electromagnetism? The concept of coming before (cause and effect) only comes into existence once tangles are complex enough to compose the concept. But, it is an approach to understanding not necessarily understanding.
What is gravity? A special case of attraction between tangles that can be replaced by appropriate equations for electromagnetism including such forms as the strong force and weak force.
Does the universe have a beginning? The answer is unknown because a beginning may be created by tangles just as an end may be created.
Is the universe rational? It is irrational resulting in irrational real numbers such as π. The imaginary number i = (-1)1/2 does not exist in the universe but does exist in hominin mathematics.
What moves through the speed of light? As the universe of electromagnetism is uncountable a method or technique for propelling or motivating an object to move across the universe is developable.
Cratering laboratory
Electric orbits
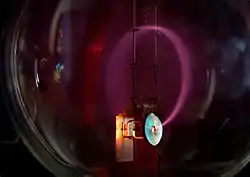
This laboratory is an activity for you to calculate an electric or magnetic orbit of an astronomical object. While it is part of the astronomy course principles of radiation astronomy, it is also independent.
Some suggested entities to consider are electric fields, magnetic fields, mass, charge, Euclidean space, Non-Euclidean space, or spacetime.
Okay, this is an astronomy orbits laboratory, specifically to try out electric/magnetic orbits and where possible compare them to those calculated using gravity.
Yes, this laboratory is structured.
I will provide an example of an electric/magnetic orbit. The rest is up to you.
Questions, if any, are best placed on the discussion page.
Electric orbits control group
The findings demonstrate a statistically systematic change from the status quo or the control group.
For an electric or magnetic orbit, what would make an acceptable control group? Think about a control group to compare your calculations to.
I will provide one for my example.
Elliptical orbit
In terms of Cartesian coordinates, the general equation in three dimensions (x,y,z) for an object moving through the solar system is
In terms of right ascension (RA) and declination (Dec), the Cartesian coordinates become
To recover the Cartesian coordinates
Sources of forces
The Lorentz force is the combination of electric and magnetic force on a charge due to both fields.
For a particle of charge q moving with a velocity v through an electric field Ef and a magnetic field B, there is a force. There is an opposite reactive force. In the case of the magnetic field:
The gravitational force is given by
- ,
where:
- Fg is the force between the masses,
- G is the gravitational constant,
- m1 is the first mass,
- m2 is the second mass, and
- r is the distance between the centers of the masses.
Object approach
Consider an object of mass (m = 0.1M⊙) passing through the heliosphere of the Sun at a velocity of 20 km s-1 from a galactic location originally outside the heliosphere. There are no other large objects in orbit around the Sun at the time of this object's entry. Jupiter, Saturn, Uranus, and Neptune are not present.
The object has a surface negative charge of 0.2Q⊙ and an intrinsic magnetic field of 100 Gauss (G) directed vertically. A current of 5 e- per second is following along magnetic field lines toward the Sun from the object. A comparable number of protons are following magnetic field lines from the Sun to the object.
At t0 the object is at 1,000 AU. Its RA is 14h 30m Dec +46° 30'.
Let the Sun have a magnetic field of 1 G directed vertically. The charge on the Sun (Q⊙) is -3 x 1027 e.s.u.
Gravity
The apparent forces on the Sun and the object are
- ,
where:
- Fg is the force between the masses,
- G is the gravitational constant,
- 0.1M⊙ is the first mass,
- M⊙ is the second mass, and
- r is the distance between the centers of the masses.
Conversion of units:
- 1M⊙ ≈ 2 x 1030 kg
- 1 AU ≈ 1.5 x 108 km
- G ≈ 6.67 x 10-17 Nˑ(km/kg)2
Replacing the symbols with their physical equivalents yields
But, each of the constants used are approximates so Fg ≈ 1.19 x 1021 N.
Electrostatics
Coulomb's law states that the electrostatic force experienced by a charge, at position , in the vicinity of another charge, at position , in vacuum is equal to:
where is the electric constant or the permittivity of free space and is the distance between the two charges and the constant is in SI units of C2 m−2 N−1, where C is Coulombs.
ε0 ≈ 8.854 x 10-12 C2 m−2 N−1, or
ε0 ≈ 8.854 x 10-12 C2 10-6 km−2 N−1, then
ε0 ≈ 8.854 x 10-18 C2 km−2 N−1.
1 e.s.u. ≈ 3.34 x 10-10 C.
Each of the constants used are approximates so Fq ≈ 8.02 x 1028 N.
Magnetostatics
If the velocity noted on approach is the vector component pointed toward the Sun, then the angle between the velocity vector and the magnetic field of the Sun is about 180°.
Approximately, FqB ≈ 4.01 x 1017 N.
The total Lorentz force is predominantly the electrostatic force.
Statuses
The Larmor radius is the radius of the circular motion of a charged particle in the presence of a uniform magnetic field.
“[F]or a particle of energy E in EeV and charge Z in a magnetic field B in µG [the Larmor radius (RL)] is roughly”[2]
where
- is the Larmor radius,
- is the energy of the particle in EeV
- is the charge of the particle, and
- is the constant magnetic field.
Or,
where
- is the Lamor or gyroradius,
- is the mass of the charged particle,
- is the velocity component perpendicular to the direction of the magnetic field,
- is the charge of the particle, and
- is the constant magnetic field for the Sun.
Larmor radii
For the object, as deflected by the Sun,
Or,
For the Sun, as deflected by the object,
Or,
Escape velocity
For a spherically symmetric body, the escape velocity at a given distance is calculated by the formula:[3]
where G is the universal gravitational constant (G = [6.67×10−17 km2 kg−2 N]), M the mass of the planet, star or other body, and r the distance from the center of gravity. The value GM is called the standard gravitational parameter, or μ, and is often known more accurately than either G or M separately.
The escape velocity for the Sun at 1,000 AU is
The escape velocity for the object at 1,000 AU is
Pauli's exclusion principle
Another possible concern is Pauli's exclusion principle that incoming charged particles of the same sign must have opposite spins.
Initial position
At initial detection
- the Lamor radius is 1.33 x 10^9 AU,
- the escape velocity of the Sun is 1.33 km/s at 1,000 AU,
- the escape velocity of the object is 0.422 km/s at 1,000 AU.
Forming an orbit
In order for the object to have an orbit around the Sun, its approach velocity of 20 km/s must be reduced to below the Sun's escape velocity of 1.33 km/s.
The electrostatic force is the major available force of repulsion to slow down the object. As it continues to travel closer to the Sun, the forces increase. But, an estimate can be calculated of how much time is required to reduce the object's velocity to below the Sun's escape velocity.
Or,
Electric orbit evaluation
To assess your orbit calculations, including your justification, analysis and discussion, I will provide such an assessment of my example for comparison.
Title is "Back of the Envelope Electric Orbit".
by Marshallsumter (discuss • contribs) 22:49, 21 January 2014 (UTC).
Abstract
Using elementary formula an object's approach to the Sun is described using gravity and the Lorentz force. As both the Sun and the object have charges, the possible Larmor radii are calculated. Each object has an escape velocity with respect to the other. With an initial velocity toward the Sun it is shown that the overwhelming force is the electrostatic repulsion between the Sun and the object. Should this force continue to operate as the object moves toward the Sun, after more about 12.9 hours the object's velocity will be below the escape velocity of the Sun. Whether the object's orbit is stable may depend on any likely tangential velocity. If there is none the object will eventually be repelled back out of the heliosphere. As there are currents of electrons and positive charges flowing between the two objects, the electrostatic repulsion could be reduced until only gravity holds the object in an orbit around the Sun.
Introduction
The objective is to test whether the measured charge on the Sun and a similar astronomical object entering the heliosphere would be sufficient to slow the object down sufficiently to allow its incoming velocity to drop below the Sun's escape velocity. In the past only gravity has been considered, with the result that the Sun cannot capture another astronomical object into an orbit unless its incoming velocity is less than 0.5 km/s and another object such as Jupiter assists.
Experimentation
Using an object of mass 10 % of the Sun's and 20 % of the Sun's charge with an incoming velocity toward the Sun of 20 km/s several calculations were made of the instantaneous forces between the objects at the initial approach.
Larmor radii were calculated to show the likely deflection of each object by the other. The escape velocities were also calculated as a simple way of assessing whether the forces involved could brake the object sufficiently to allow capture.
Discussion
The possible effect of the Pauli exclusion principle was not assessed. The forces were not allowed to vary differentially as the object continued to approach which would likely reduce the time to capture. If no tangential velocity exists the object would eventually be repelled back out of the solar system. Charge dissipation was not considered even though initial currents were indicated. Increases in either currents may have mitigated the repulsion and either sped up or slowed down the capture.
Conclusion
Although all likely conditions were not considered, a back-of-the-envelope calculation suggests that an object entering the heliosphere with a charge comparable to that of the Sun, but at 40 times the currently accepted incursion and capture velocity, could be captured into some kind of orbit. The stability of the orbit with time was not assessed.
Electron beam heating laboratory
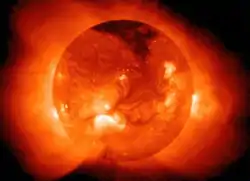
This laboratory is an activity for you to create a method of heating the solar corona or that of a star of your choice. While it is part of the astronomy course principles of radiation astronomy, it is also independent.
Some suggested entities to consider are electromagnetic radiation, electrons, positrons, neutrinos, gravity, time, Euclidean space, Non-Euclidean space, magnetic reconnection, or spacetime.
More importantly, there are your entities.
Please define your entities or use available definitions.
Usually, research follows someone else's ideas of how to do something. But, in this laboratory you can create these too.
Okay, this is an astronomy coronal heating laboratory.
Yes, this laboratory is structured.
I will provide an example of electron beam heating calculations. The rest is up to you.
Questions, if any, are best placed on the discussion page.
Electron beam heating control group
The findings demonstrate a statistically systematic change from the status quo or the control group.
For creating a heating method, what would make an acceptable control group? Think about a control group to compare your universe or your process of creating a universe to. Or, at least a control group to compare your heating technique to.
I will provide one example.
Electron beam heating
The lecture electron beam heating describes at least one example of the use of this technology to heat objects.
Fluxes
Def. the "rate of transfer of energy (or another physical quantity) through a given surface, specifically electric flux, magnetic flux"[4] is called a flux.
"Mass flux of species α [is in units of] g/cm2 s"[5] or, in dimensions [quantity of species α]·[area]−1·[time]−1, where an area may be cm2.
Detector distances
Let Voyager 1 be 17,932,000,000 km (119.9 AU) from the Sun at RA 17.163h Dec +12.44°, ecliptic latitude of 34.9°.
Let Voyager 1, now headed out of the solar system, be traveling at 17 km/s (38,000 mph).
At this distance, the Voyager 1 electron detector has detected both the number and direction of extraheliosphere electrons. For this laboratory example, let the electron flux be 2 e- cm-2 s-1 diffusing into our solar system from elsewhere in the galaxy. Each of these electrons has an energy of 10 MeV.
Electron beams
As of December 5, 2011, "Voyager 1 is about ... 18 billion kilometers ... from the [S]un [but] the direction of the magnetic field lines has not changed, indicating Voyager is still within the heliosphere ... the outward speed of the solar wind had diminished to zero in April 2010 ... inward pressure from interstellar space is compacting [the magnetic field] ... Voyager has detected a 100-fold increase in the intensity of high-energy electrons from elsewhere in the galaxy diffusing into our solar system from outside ... [while] the [solar] wind even blows back at us."[6]
Surface areas
From the image at the top of this laboratory it appears that the coronal clouds about the Sun cover a partial shell from say 80° N to about 80° S that follows the curvature of the photosphere. There does seem to be a physical separation of the shell of coronal clouds from the concentric shell of the photosphere. The coronal holes perhaps being void of incoming electron flux.
Photosphere volume
R⊙eq ≈ 6.955 x 105 km. The thickness of the photosphere is about 400 km. R⊙p ≈ 6.951 x 105 km.
Photosphere hydrogen
The density of the Sun is about 2 x 10-4 kg m-3. Or,
One mole of H2 (gas) has a mass of 2.016 x 10-3 kg. The molar density of the photosphere may be
Constant volume specific heat capacity
For H2 (gas) the molar constant-volume heat capacity at 298 K is 20.18 J/(mol · K). At 2000 K it is about 25 J/(mol · K). Using a linear extrapolation,
for 5777 K, yields
Before calculating the amount of energy or power necessary to heat the coronal clouds around the Sun, let's see if the influx of electrons from outside the heliosphere may be able to heat the surface of the photosphere (p) to 5777 K from 100 K.
Photosphere heating
Voyager 1 is 17,932,000,000 km (119.9 AU) from the Sun at RA 17.163h Dec +12.44°, ecliptic latitude of 34.9°.
For this laboratory example, let the electron flux be 2 e- cm-2 s-1 diffusing into our solar system from elsewhere in the galaxy. Each of these electrons has an energy of 10 MeV.
If the electron flux measured by Voyager 1 is close to 2 e- cm-2 s-1 where each electron averages 10 MeV and these electrons are heading for the Sun, then each electron may strike the photosphere from anywhere in a sphere around the Sun.
To heat the photosphere to 5777 K takes
The power (P) that may be deposited on the photospheric surface of the Sun is
The luminosity (in Watts, W) of the Sun is 3.846 x 1026 W. In eV/s this is
If the energy of the incoming electrons is 700 MeV and the flux is 8.48 x 104 e- cm-2 s-1, then the power from the incoming electrons would be
Coronal heating
The power to heat the solar corona is on the order of 1039 eV s-1. While this is within range of the estimated electron influx beam heating, X-rays are usually generated between 0.1 and 120 keV. 10 to 700 MeV electrons would likely produce gamma rays rather than X-rays.
Electron beam heating report
Title: Corona and photosphere heating by interstellar electron influx.
Author: Marshallsumter (discuss • contribs) 01:08, 31 January 2014 (UTC)
Abstract:
By using estimates of the interstellar electron influx that may have been measured by Voyager 1, a back-of-the-envelope calculation shows that the electron influx may be sufficient to heat the solar corona to MK and the photosphere to 5777 K. These estimates put the coronal heating problem in perspective of the overall external heating of the outer Sun including the photosphere.
Introduction:
The news report of Voyager 1 reaching some 120 AU from the Sun contain interesting discoveries. The most significant may be a flux of electrons diffusing into our solar system from elsewhere in the galaxy. The solar wind has ceased earlier and has even been turned backward. These results suggest a net influx of electrons perhaps toward the Sun that may be involved in heating the solar corona. The possibility is explored using some "back-of-the-envelope" calculations.
Experimentation:
The experiment consists of two parts: the amount of power to either heat the photosphere to an effective temperature of 5777 K or to at least match the Sun's luminosity and to heat the solar corona into the MK range so that X-rays are emitted.
To accomplish the first experiment the volume of the photosphere is calculated followed by the number of moles of diatomic hydrogen gas, the principal component of the photosphere. Using an estimated constant volume specific heat capacity the amount of heat needed to warm the Sun up from 100 K (a likely pre-luminescent temperature of the proto-Sun before fusion to 5777 K.
The second experiment is accomplished by estimating the power and influx of electrons from elsewhere in the galaxy.
Discussion:
The power calculated for the electron influx is compared with the first experiment and with the known luminosity of the Sun.
The initial guess put the power at about six orders of magnitude too low to match the Sun's current luminosity. However, increasing the electron energy by 70 times and the influx by about 4 x 104 brings the estimate into agreement. It is likely that either increase may be too much.
Other concerns focus on whether any of these electrons can reach the Sun. The Sun has a net surface negative charge. The solar wind contains electrons, protons and heavier nuclei that may alter the energy or influx significantly.
Conclusions:
While likely factors exist that may decrease the estimated electron energy or influx, it appears that such an influx may not only heat the solar corona but also the photosphere. Actual numbers from Voyager 1 may shed more light on this conclusion.
Electron beam heating evaluation
To assess your method of heating the solar corona, including your justification, analysis and discussion, I will provide such an assessment of my example for comparison.
If you chose another star, even of a different spectral type, be sure to include likely scale factors or other adjustable parameters.
Evaluation
Unless the influx is significantly higher at lower energies it seems that 10 to 700 MeV is way too high. The corona of the Sun would be producing gamma-rays not X-rays. No justification is given for the 104th increase in the influx estimate. Actual data on interstellar electrons does suggest MeV levels.
Galaxies laboratory
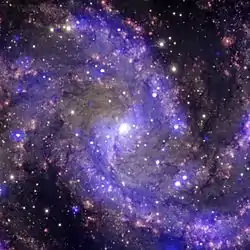
This laboratory is an activity for you to create or define a galaxy. While it is part of the astronomy course principles of radiation astronomy, it is also independent.
Galaxy verification
According to NASA,[7] "NGC 6946 is a medium-sized, face-on spiral galaxy about 22 million light years away from Earth. In the past century, eight supernovas have been observed to explode in the arms of this galaxy. Chandra observations (purple [in the image at the top right of the resource]) have, in fact, revealed three of the oldest supernovas ever detected in X-rays, giving more credence to its nickname of the "Fireworks Galaxy." This composite image also includes optical data from the Gemini Observatory in red, yellow, and cyan."
But is it?
Orientation
The composite image does appear to conform to a face-on galaxy.
Symmetry
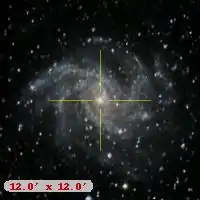
From question 1 of the galaxies/Quiz and the image at the top of this laboratory, NGC 6946 does not appear to fall easily into any of the six forms of rotational symmetry having about 5 spiral arms on the left and maybe 2 on the right.
Further, the image in this section from SIMBAD appears to have two-fold rotational symmetry with three spiral arms on each side.
Morphology
The primary source used by SIMBAD considers the nebula to be one of 30 nearby spiral galaxies.[8]
"Targets span a wide range in Hubble type, star formation activity, morphology, and inclination."[8]
Distances
Distance moduli have been estimated for NGC 6946 using its brightest blue stars and its HII ring.[9] Its distance modulus is estimated to be log D0 = 4.434.[9] The distance in parsecs is given by
where µ0 = 29.25.[9] NGC 6946 is at 106.85 (7.08 x 106) parsecs, or approximately 23.1 x 106 light years. While this is greater than the NASA number, it is not an order of magnitude greater of smaller.
Classifications
In the galaxies lecture is a composite image of Hubble's classification scheme for galaxies. Using this as a visual guide and examining the multispectral image at the top of this resource, NGC 6946 appears to be close to type Sb (example, NGC 2841).
Looking up "NGC 6946" on SIMBAD, without the quotes, reveals that SIMBAD considers NGC 6946 to be an "HII Galaxy" of morphological type "SAB(rs)cd".
A more extensive classification scheme starting from the Hubble scheme indicates that an Sab galaxy is approximately in between Sa and Sb.[10]
Special characteristics
H II ring(s) and/or regions have been noted.[9]
Galaxies report
Title: Evaluation of nebula NGC 6946.
Author: --Marshallsumter (discuss • contribs) 00:19, 9 February 2014 (UTC)
Abstract:
Starting with a nebula classification scheme developed by Edwin Hubble in 1926, a morphological assessment of the nebula NGC 6946 has been made. From observational structure recorded on two independent images, NGC 6946 appears to be a face-on spiral galaxy of type Sab at approximately 23 million light years.
Introduction:
In many nebula images and photographs it is often difficult to determine whether stars or relatively diffuse gaseous or molecular clouds are being imaged. Using additional observations and deductions, even if qualitative, previous claims regarding nebula NGC 6946 are investigated.
Experimentation:
Although nebula NGC 6946 is not known to rotate during observation in the plane of view or vertical to it, its appearance in both images is assessed using the Hubble scheme and forms of rotational symmetry described in question number 1 of galaxies/Quiz. Orientation is determined from image structure and symmetry.
Additional individuals have recorded their opinions on morphology and an assessment based on the Hubble scheme has been estimated.
A distance calculation from 1978 based on secondary indicators has been made.
Several primary sources are consulted regarding classification and special characteristics.
Discussion:
Earlier orientational analyses confirm that the nebula is a face-on galaxy.
Close-up symmetry analysis suggests that a composite image of the nebula from several spectral ranges has no rotational symmetry yet appears spiral-like in morphology.
Distance calculations and gross classifications appear to be supported by independent primary sources.
Special characteristics of H II ring(s) or regions are confirmed by at least one primary source.
Conclusions:
Although NGC 6946 has not apparently moved in its orientation over recent human observations, the nebula appears to be a face-on spiral galaxy of type Sab or Sb at approximately 23 million light years.
Galaxies evaluation
The finer notations of galaxy classification "(rs)cd" have not been examined or explained. Other independent radiation astronomies have not been consulted for images of the nebula. While several primary authors report stars at great distances indicating a galaxy rather than a spiral star cluster within the Galaxy, individual representations to show this to be the case have not been directly presented or evaluated.
Intergalactic medium laboratory
.jpg.webp)
This laboratory is an activity for you to explore the universe for an intergalactic medium. While it is part of the astronomy course principles of radiation astronomy, it is also independent.
Interaction degree
In the lecture intergalactic medium, interacting galaxies are introduced. For my example, I will attempt to evaluate the degree of interaction of the two galaxies in the image at the top of this resource. If you want to evaluate a pair of interacting galaxies, here is a fairly current list from the category at Wikimedia Commons: interacting galaxies.
Galaxies introduction
"UGC 9618, also known as VV 340 or Arp 302 consists of a pair of very gas-rich spiral galaxies in their early stages of interaction: VV 340A is seen edge-on to the left, and VV 340B face-on to the right. An enormous amount of infrared light is radiated by the gas from massive stars that are forming at a rate similar to the most vigorous giant star-forming regions in our own Milky Way. UGC 9618 is 450 million light-years away from Earth, and is the 302nd galaxy in Arp's Atlas of Peculiar Galaxies."[11] The image was taken through the Hubble Space Telescope F435W (B) and F814W (I) filters for 33 min of exposure. The estimated distance is 450 million light-years.
Galaxies experimentation
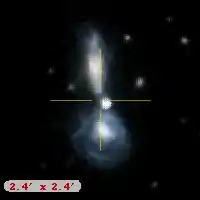
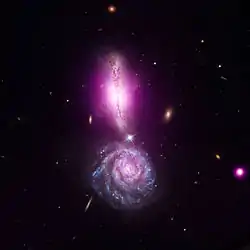
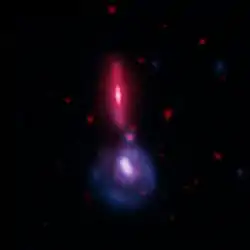
The coordinates for UGC 9618 are 14h 57m 00.4s +24° 36' 44" at the center of the cross hairs.
According to SIMBAD VV340A is the face-on galaxy, and VV340B is the edge-on galaxy.
In both images of UGC 9618, 50 mm corresponds to 2.4' of arc. The galaxy on edge is 16 mm in length, while the other is closer to 12 mm. At 4.5 x 108 lyrs, VV340B (edge-on) is about 1.01 x 105 lyrs in diameter, while VV340A (face-on) is about 7.55 x 104 lyrs in diameter.
VV340A (SIMBAD) appears to be Hubble sequence Sa type galaxy, with VV340B (SIMBAD) may be similar. VV340A (SIMBAD) is a right-handed spiral, with its axis pointed out of the paper plane toward the viewer (face-on).
VV340A (SIMBAD) has its center closer to the point between the two (4 mm) than to the opposing edge (8 mm) suggesting that its spiral arms on the side closest to VV340B (SIMBAD) are missing or compressed by about 50 % compared to the opposing side. This suggests that VV340A (SIMBAD) has loss less than 33 % of its luminous sources either to VV340B (SIMBAD), to the interaction between them, or to compression in the direction of VV340A (SIMBAD).
VV340B (SIMBAD) appears to have its center at 7 mm from the interaction volume whereas the other side away from the interaction is about 9 mm in width. This suggests only about 13 % or less of VV340B (SIMBAD) has been lost to the interaction, VV340A (SIMBAD), or compression.
The bright luminous source in the interaction volume may be a foreground star or a supernova in the interaction volume.
Additional images of UGC 9618 are available at NED: Images and maps in NED archive for object UGC 09618.
If the interaction is endothermic (absorbs energy), then luminous sources in or near the interaction volume may be no longer visually luminous (stars may have gone out).
If the interaction is exothermic (gives off energy), then the apparently missing luminous sources may now be radiating in the ultraviolet or shorter wavelengths such as X-rays.
Neither SIMBAD nor NED shows either galaxy as an X-ray source, but both are infrared sources.
The image at right shows VV340B (SIMBAD), the northern (N) galaxy as a major source of X-rays (purple). VV340A (SIMBAD), the southern galaxy (S), is in red, green, and blue.
In the second image at right, the northern galaxy VV340B is primarily an infrared image, whereas VV340A is primarily an ultraviolet image.
Galaxies results
The lack of high energy output from the interaction volume suggests that any loss of luminous sources from either galaxy is not exothermic. The lack of equal or excessive endothermic energy suggests that luminous sources are not being lost due to interaction. The asymmetry in both galaxies may indicate a common origin originally between them. Both galaxies have removed luminous sources from the interaction volume.
Galaxies discussion
The visual/optical image at the top of the resource shows the edge-on galaxy as yellow to orange in color with prominent dust lanes. The face-on galaxy seems more bluish suggesting younger stars.
Both the X-ray image and the optical/visual image suggest that the edge-on galaxy is closer to a Hubble type S0 than any other type.
The lack of luminous sources between the galaxies in the interaction volume does not suggest that these galaxies are merging.
The asymmetry in both galaxies, primarily toward the interaction volume, may indicate a common origin originally between them. Both galaxies have removed luminous sources from the interaction volume and may be actually moving apart from their common and competitive origin.
The asymmetry of both galaxies roughly centered on the interaction volume argues against these two galaxies being double galaxies (one nearer than the other, but not interacting) rather than interacting galaxies.
Galaxies conclusions
The pair of galaxies that compose UGC 9618 appear to be interacting rather than a mere galaxy double. The lack of luminous sources at any wavelength in the interaction volume suggests that both galaxies have cleaned out nearly all luminous sources from the interaction volume. The asymmetry being approximately centered on the interaction volume together with the lack of luminous sources within this volume suggests that the two galaxies are not merging but separating.
Galaxies report
Title
The degree of interaction in the UGC 9618 galaxy system
by --Marshallsumter (discuss • contribs) 23:14, 15 February 2014 (UTC)
Abstract
The pair of galaxies that compose UGC 9618 are analyzed with respect to their degree of interaction. VV340A appears to be less than 33 % involved in the interaction, while VV340B is less than or about 13 % involved. The distribution and radiation types of luminous sources suggests that the two galaxies are separating or at least not merging from a possible common origin.
Introduction
Current opinion is that VV340A and VV340B are in the early stages of merging. "An enormous amount of infrared light [from VV340B] is radiated by the gas from massive stars that are forming at a rate similar to the most vigorous giant star-forming regions in our own Milky Way."[11]
Experiment
Measurements are made of the approximate distribution of luminous sources in both galaxies. Images of UGC 9618 from various radiation astronomies are checked for information regarding either galaxy. Scans of these galaxies are available from microwave astronomy through X-ray astronomy.
Results
SIMBAD does not report the bright luminous source apparently in the interaction volume as a supernova so it is likely a foreground star or a star cluster remnant in the interaction volume. Orange and yellow astronomy reveal that the edge-on galaxy may be composed of older or cooler stars. The prevalence of blue (from blue astronomy) in the face-on galaxy suggests younger or hotter stars visually. Ultraviolet astronomy of the face-on galaxy also suggests hotter or younger stars. Somewhat surprising is the enormous amount of X-rays from the edge-on galaxy. Usually, ultraviolet, X-rays and gamma-rays are associated with very hot sources.
Measurements of the distribution of luminous sources suggest that an asymmetry in both galaxies exists toward and with respect to the interaction volume.
With the exception of the one very bright source on the edge of the interaction volume, no luminous sources of any kind exist between the two galaxies in the interaction volume.
Discussion
The infrared portion of the composite image with ultraviolet strongly suggests that the edge-on galaxy is much cooler in general than the face-on galaxy.
The large X-ray output surrounding primarily the more central portion of the edge-on galaxy suggests a very high temperature galactic coronal cloud. X-rays are detected from both galaxies though mostly only from the inner core region of the face-on galaxy much like the Milky Way.
The lack of luminous sources between the galaxies in the interaction volume suggests that these galaxies are not merging.
The asymmetry in both galaxies, primarily toward the interaction volume, may indicate a common origin originally between them. Both galaxies have removed luminous sources from the interaction volume and may be actually moving apart from their common and competitive origin.
The asymmetry of both galaxies roughly centered on the interaction volume argues against these two galaxies being double galaxies (one nearer than the other, but not interacting) rather than interacting galaxies.
Conclusion
The pair of galaxies that compose UGC 9618 appear to be interacting rather than a mere galaxy double. The lack of luminous sources at any wavelength in the interaction volume suggests that both galaxies have cleaned out nearly all luminous sources from the interaction volume. The asymmetry being approximately centered on the interaction volume together with the lack of luminous sources within this volume suggests that the two galaxies are not merging but separating.
Galaxies evaluation
Although many radiation astronomy images of the galaxy pair UGC 9618 have been consulted, the overall conclusion that these two galaxies are not merging is in direct disagreement with several primary sources. These sources need to be consulted and examined to obtain a more thorough understanding of this galaxy system.
Locating the Sun laboratory
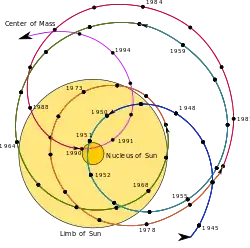
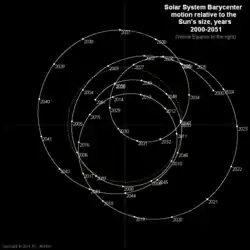
This laboratory is an activity for you to create a method for locating the Sun. While it is part of the astronomy course principles of radiation astronomy, it is also independent.
You are being asked to find a way to quantitatively and directly locate the Sun relative to a familiar Earth location.
Introduction
Generally, the Sun is not transparent to any known wavelength of electromagnetic radiation. Metal atoms in the photosphere prevent most wavelengths of radiation from traveling through the Sun. A laser oriented on the surface of Mercury to transmit a beam to the Earth, even when the Sun occults Mercury relative to Earth, would not work.
Experimentation
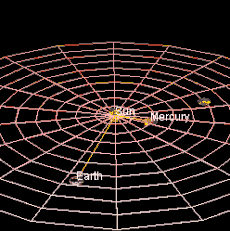
As the animation at right suggests, if Earth and Mercury were coplanar in their orbits around the Sun, Mercury would transit across the Sun at least three times in an Earth year.
This can also be directly derived from the known synodic period for Mercury with the Earth of 115.88 d.[12] The Sun could then be approximately located by the intersection of the three rays from Earth through Mercury into the Sun. As long as the three rays are extended far enough past their mutual intersection, this intersection would be the location of the Sun.
Coplanarity

Although the eccentricity of Mercury's orbit may change the coplanar transit events, the actual reason for the rarity of transits is the lack of coplanarity. The figure at right shows the approximate locations of the poles of the various planet orbits.
Mercury's orbital pole is closest to the Sun's north pole, while Earth's is in the grouping above and to the right of Mercury's.
Historical transits
Transits of Mercury | |||||
---|---|---|---|---|---|
Date of mid-transit | Time (UTC) | Notes | Images | ||
Start | Mid | End | |||
743 Oct 28 | |||||
1342 Oct 21 | |||||
1631 Nov 7 | Observed by Pierre Gassendi. | ||||
1651 Nov 3 | Observed by Jeremy Shakerly in Surat, reported in letter to Henry Osbourne, January 1652. Shakerly is thought to have died in India around 1655.[13] | ||||
1661 May 3 | Occurred on the day of the Coronation of King Charles II of England. Observed by Christiaan Huygens in London. | ||||
1677 Nov 7 | Observed by Edmund Halley in St Helena, Richard Towneley in Lancashire, Jean Charles Gallet in Avignon; as reported in letter from John Flamsteed to Johannes Hevelius 23 May 1678 [14] | ||||
1743 Nov 5 | Coordinated scientific observations were organized by Joseph-Nicolas Delisle worldwide. | ||||
1753 May 6 | |||||
1769 Nov 9 | 23:09 | Observed by Charles Green and James Cook from Mercury Bay in New Zealand.[15] Noted that Mercury had little or no atmosphere. | |||
1802 Nov 9 | 06:16 | 08:58 | 11:41 | ||
1815 Nov 12 | 00:20 | 02:33 | 04:46 | ||
1822 Nov 5 | 01:04 | 02:25 | 03:45 | ||
1832 May 5 | 09:04 | 12:25 | 15:47 | ||
1835 Nov 7 | 17:35 | 20:08 | 22:41 | ||
1845 May 8 | 16:24 | 19:37 | 22:49 | ||
1848 Nov 9 | 11:07 | 13:48 | 16:28 | ||
1861 Nov 12 | 05:21 | 07:19 | 09:18 | ||
1868 Nov 5 | 05:28 | 07:14 | 09:00 | ||
1878 May 6 | 15:16 | 19:00 | 22:44 | ||
1881 Nov 7 | 22:19 | 00:57 | 03:36 | ||
1891 May 9 | 23:57 | 02:22 | 04:47 | ||
1894 Nov 10 | 15:58 | 18:35 | 21:11 | ||
1907 Nov 14 | 10:24 | 12:07 | 13:50 | ||
1914 Nov 7 | 09:57 | 12:03 | 14:09 | ||
1924 May 8 | 21:44 | 01:41 | 05:38 | ||
1927 Nov 10 | 03:02 | 05:46 | 08:29 | ||
1937 May 11 | 08:53 | 08:59 | 09:06 | Only visible as partial transit in Southern Africa, Southern Arabia, South Asia and Western Australia. | |
1940 Nov 11 | 20:49 | 23:21 | 01:53 | ||
1953 Nov 14 | 15:37 | 16:54 | 18:11 | ||
1957 May 6 | 23:59 | 01:14 | 02:30 | ||
1960 Nov 7 | 14:34 | 16:53 | 19:12 | [16] | |
1970 May 9 | 04:19 | 08:16 | 12:13 | [17] | ![]() |
1973 Nov 10 | 07:47 | 10:32 | 13:17 | [18] | ![]() |
1986 Nov 13 | 01:43 | 04:07 | 06:31 | [19] | |
1993 Nov 6 | 03:06 | 03:57 | 04:47 | [20] | |
1999 Nov 15 | 21:15 | 21:41 | 22:07 | [21] Partial transit in Australia, Antarctica and New Zealand's South Island | |
2003 May 7 | 05:13 | 07:52 | 10:32 | [22] | ![]() |
2006 Nov 8 | 19:12 | 21:41 | 00:10 | [23] | ![]() |
Synodic period
Using the last visible transit of 2006 11 08, and an approximate synodic period of 116 d, the two previous passes of Mercury occurred on 2006 07 15 and 2006 03 21. On these two previous days there is no direct observation of Mercury in front of the Sun by line of sight, but the dates are based on observations of the synodic period.
To verify the synodic period, the last three visible transits should be approximate multiples of the period.
Transit date | Number of days | Synodic periods | Closest pass | Days to pass | Number of periods | Needed days |
---|---|---|---|---|---|---|
1999 11 15 | 0 | 0 | 1999 11 15 | 0 | 0 | 0 |
2003 05 07 | 1269 | 10.9 | 2003 05 07 | 15+31+366+365+365+31+28+31+30+7 | 10.95 | +6 |
2006 11 08 | 2550 | 22.006 | 2006 11 08 | 366+365+365+24+30+31+31+30+31+8 | 22.006 | -1 |
The synodic period varies by about ±3.5 d.
The three rays
The three rays even without visible transits of Mercury across the Sun should have occurred on 2006 03 21, 2006 07 15, and 2006 11 08.
Relative to the fixed background stars, each of these days has a specific right ascension. The first occurs 80 d into the year so (80 d/365 d)*24 h = 5h 15m 37s. The second occurs 196 d into the year or (196 d/365 d)*24 h = 12h 53m 16s. The third occurs 312 d into the year or (312 d/365 d)*24 h = 20h 30m 54s.
The ecliptic for each of these rays should be in Taurus, Virgo, and Capricornus, respectively. The declinations are approximately +23.2°, -5.0°, and -18.5°.
In summary, the three rays whose approximate intersection or closest pass locates the Sun within a small volume are
- RA 5h 15m 37s Dec +23.2°, with a range of RA 5h 01m 48s Dec +23.2° to RA 5h 29m 25s Dec +23.2°,
- RA 12h 53m 16s Dec -5.0°, with a range of RA 13h 07m 4s Dec -5.0° to RA 12h 39m 27s Dec -5.0°, and
- RA 20h 30m 54s Dec -18.5°, with a range of RA 20h 44m 43s Dec -18.5° to RA 20h 17m 6s Dec -18.5°.
Intersection volume
The measurement of the solar parallax would use
The actual distance between each measurement is the chord between the two measurement points rather than 1 AU. This displacement (d1, d2, or d3) is given by
where is the angle between the first and second passes.
Using the Universal coordinate converter to obtain the angle between passes yields 114.38°, 111.27°, and 134.34°. d1 = 0.8405 AU, d2 = 0.8255 AU, and d3 = 0.9217 AU.
Locating the Sun results
The ranges for the angles then yield the approximate intersection volume for the Sun's location.
The distance to the Sun varies for the error (standard deviation) in d1 from 0.9829 to 1.019 AU.
The distance to the Sun varies for the error in d2 from 0.9818 to 1.020 AU.
The distance to the Sun varies for the error in d3 from 0.9889 to 1.012 AU.
These suggest that the position of the Sun along each ray varies from about 0.9845 to 1.017 AU, or at the extreme limits for the standard deviation in the synodic period from 0.9818 to 1.020.
Locating the Sun discussion
For an AU = 1.496 x 108 km, the extremes from these back-of-the-envelope calculations suggest that because of the variations in the synodic period of Mercury the intersection or location volume of the Sun may vary from 0.9818 (1.469 x 108 km) to 1.020 (1.526 x 108 km. The location of the Sun may vary from about 2.7 million to 3.0 million kilometers during a given year, or about 2 %, from a central position.
The durations of passes or transits may be upwards of 8 h. This suggests that the variations in the synodic period relate directly to the variations of the orbit of Mercury. While the Sun may move throughout the year, including changes in its diameter, there is no direct evidence that the Sun is outside Mercury's orbit (about 50 million kilometers in diameter) at any time.
A greater number of transits would likely reduce the error on the Sun's location using line of sight.
The mean diameter of the Sun's photosphere is 1,392,684 km. The large variations suggested here would amount to more than four solar radii in either direction from a possible central position. It may be the case that the coronal cloud around the Sun affects Mercury's orbit regarding its synodic period.
Locating the Sun conclusions
A series of elementary back-of-the envelope calculations has verified using recent transits of Mercury between the Sun and the Earth that the location of the Sun is well within the orbit of Mercury and probably within 2 % of a central position. At the extreme the position of the Sun may be well away from the barycenter for the solar system.
Locating the Sun report
Title
The location of the Sun using Mercury Transits
by --Marshallsumter (discuss • contribs) 23:44, 22 February 2014 (UTC)
Abstract
A series of simple back-of-the-envelope calculations starting from three recent transits of the Sun by Mercury demonstrate that the Sun is highly likely to be well within the orbit of Mercury and within a few solar diameters of the solar system barycenter.
Introduction
The Sun is probably the only astronomical object in the solar system whose position cannot be verified by direct radiation astronomy. This presents certain theoretical limitations on the forces controlling the solar system. Use of transits such as Mercury between the Sun and the Earth may help to impose practical limits on the Sun's real location.
Experiment
Starting from historical records of Mercury transits and the known synodic period, a number of experiments regarding coplanarity and actual transit dates are used to calculate rays with RA and Dec that should limit the Sun's locational volume.
Results
The Sun's position along each ray tested may vary from about 0.98 to 1.12 AU.
Discussion
Limitations on the actual solar position may further refine these calculations by considering variations in Mercury's orbit and the Sun's coronal cloud.
Conclusion
The Sun's position is demonstrated with several Mercury transits to be around 2 % of the barycenter of the solar system.
Locating the Sun evaluation
Evaluation
Variations in the Earth's orbit and Mercury's orbit during 2006 which are likely available is archives or databases could have possibly reduced the uncertainty in the Sun's location.
Magnetic field reversal laboratory
Meteorites laboratory
Neutrinos from the Sun laboratory
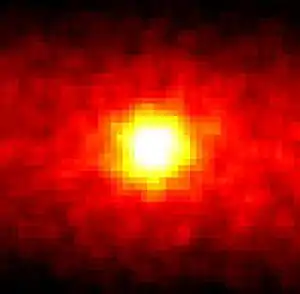
This laboratory is an activity for you to evaluate some possible origins of solar neutrinos. While it is part of the astronomy course principles of radiation astronomy, it is also independent.
Some suggested neutrino locational entities to consider are electromagnetic radiation, the neutrinos themselves, mass, time, Euclidean space, Non-Euclidean space, and spacetime.
More importantly, there are your locational or evaluative entities. And, yes, you can create as many as you need if you wish to.
You may choose to define your locational entities or use those already available.
Usually, research follows someone else's ideas of how to do something. But, in this laboratory you can create these too.
Neutrinos from the Sun proof of concept
The first concept that needs a proof of is that neutrinos can originate from some process above the photosphere within about 2-4 solar radii.
"[N]eutrino flux increases noted in Homestake results [coincide] with major solar flares [14]."[24]
This result together with those in the next two sections establishes that neutrinos are being produced by processes above the photosphere and probably within 2-4 solar radii as most solar flares give off energy close to and into the chromosphere.
Chromospheres
"The correlation between a great solar flare and Homestake neutrino enhancement was tested in 1991. Six major flares occurred from May 25 to June 15 including the great June 4 flare associated with a coronal mass ejection and production of the strongest interplanetary shock wave ever recorded (later detected from spacecraft at 34, 35, 48, and 53 AU) [15]. It also caused the largest and most persistent (several months) signal ever detected by terrestrial cosmic ray neutron monitors in 30 years of operation [16]. The Homestake exposure (June 1–7) measured a mean 37Ar production rate of 3.2 ± 1.5 atoms/day (≈19 37Ar atoms produced in 6 days) [13]; about 5 times the rate of ≈ 0.65 day −1 for the preceding and following runs, > 6 times the long term mean of ≈ 0.5 day−1 and > 2 1/2 times the highest rates recorded in ∼ 25 operating years."[24]
Coronal clouds
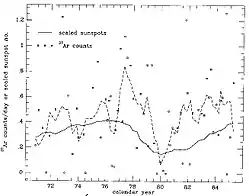
The highest flux of solar neutrinos come directly from the proton-proton interaction, and have a low energy, up to 400 keV. There are also several other significant production mechanisms, with energies up to 18 MeV.[25]
The parts of the Sun above the photosphere are referred to collectively as the solar atmosphere.[26]
"Neutrinos can be produced by energetic protons accelerated in solar magnetic fields. Such protons produce pions, and therefore muons, hence also neutrinos as a decay product, in the solar atmosphere."[27]
"Energetic protons in the solar corona could explain Figure 2 only if (1) they tap a substantial fraction of the entire energy generated in the corona, (2) the energy generated in the corona is at least 3 times what has been deduced from the observations, (3) the vast majority of energetic protons do not escape the Sun, (4) the proton energy spectrum is unusually hard (p0 = 300 MeV c-1, and (5) the sign of the variation is opposite to what one would predict. As the likelihood of all of these conditions being fulfilled seems extremely small, we do not believe that neutrinos produced by energetic protons in the solar atmosphere contribute significantly to the neutrino capture in the 37Cl experiment."[27]
Neutrinos from the Sun experimentation
In the above section five criteria for the current neutrino flux are made available to assess the neutrino flux coming from above the photosphere:
- energetic protons "tap a substantial fraction of the entire energy generated in the corona",[27]
- "the energy generated in the corona is at least 3 times what has been deduced from the observations",[27]
- "the vast majority of energetic protons do not escape the Sun",[27]
- "the proton energy spectrum is unusually hard (p0 = 300 MeV c-1",[27] and
- "the sign of the variation is opposite to what one would predict."[27]
In this section, each of these is examined using experimental results already available.
Here on the Earth's surface the νe flux is about 1011 νe cm-2 s-1 in the direction of the Sun.[28]
"The total number of neutrinos of all types agrees with the number predicted by the computer model of the Sun. Electron neutrinos constitute about a third of the total number of neutrinos. [...] The missing neutrinos were actually present, but in the form of the more difficult to detect muon and tau neutrinos."[28]
Energetic protons
The first piece of information that seems missing are the reactions that produce the higher energy neutrinos: νµ and ντ.
For antiproton-proton annihilation at rest, a meson result is, for example,
- [30] and
"All other sources of ντ are estimated to have contributed an additional 15%."[31]
for two neutrinos.[31]
where is a hadron, for two neutrinos.[31]
Coronal energy
Based on the 3He-flare flux from the Sun's surface and Surveyor 3 samples (implanted 15N and 14C in lunar material) from the surface of the Moon, the level of nuclear fusion occurring in the solar atmosphere is approximately at least two to three orders of magnitude greater than that estimated from solar flares such as those of August 1972.[32]
Although 7Be is usually assumed to have been produced by the Big Bang nuclear fusion, excesses (100x) of the isotope on the leading edge[33] of the Long Duration Exposure Facility (LDEF) relative to the trailing edge suggest that fusion near the surface of the Sun is the most likely source.[24] The particular reaction 3He(α,γ)7Be and the associated reaction chains 7Be(e-,νe)7Li(p,α)α and 7Be(p,γ)8B => 2α + e+ + νe generate 14% and 0.1% of the α-particles, respectively, and 10.7% of the present-epoch luminosity of the Sun.[34] Usually, the 7Be produced is assumed to be deep within the core of the Sun; however, such 7Be would not escape to reach the leading edge of the LDEF.
Proton escapes
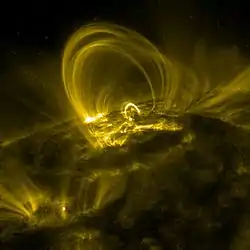
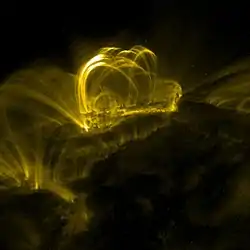
"Almost as soon as Active Region 10808 rotated onto the solar disk, it spawned a major X17 flare. TRACE was pointed at the other edge of the Sun at the time, but was repointed 6 hours after the flare started. The image on the left shows the cooling post-flare arcade (rotated by -90 degrees so that north is to the right) 6h after the flare (at 00:11 UT on September 8); the loop tops still glow so brightly that the diffraction pattern repeats them on diagonals away from the brightest spots. Some 18h after the flare, the arcade is still glowing, as seen in the image on the right (at 11:42 UT on September 8). In such big flares, magnetic loops generally light up successively higher in the corona, as can be seen here too: the second image shows loops that are significantly higher than those seen in the first. Note also that the image on the right also contains a much smaller version of the cooling arcade in a small, very bright loop low over the polarity inversion line of the region."[35]
Nearly all of the TRACE images of coronal loops and the transition region indicate that material in these loops and loop-like structures returns to the chromosphere.
"Normally, solar energetic particle (SEP) events associated with disturbances in the eastern hemisphere are characterized by slow onset and lack of high-energy particles. The SEP event associated with the first major flare (X17) [...] is among very few such events over several decades in that although the source region was on the east limb, the particle flux started to rise only a few hours from the flare onset, while the flux of protons with energies in excess of 100 MeV went up by more than a factor of one hundred. We do not understand how these energetic particles can reach the Earth from that side of the Sun, because there should be no magnetic connectivity."[35]
Hard protons
As the above sections suggest, the amount of hardness of the proton spectrum may be partially met by the accelerator-like activity of the coronal loops during most of the solar cycle.
Perhaps even more important is the strong correlation of the four near-zero neutrino detections with no scatter at the sunspot minimum. Is it the case that few if any neutrinos are produced at sunspot minimum or solar cycle minimum?
Signs of variation
While more information is needed, it may be the case that both νµ and ντ need not be oscillation products but may occur with or without oscillation as a normal decay product. Initial detection of these other two neutrinos help to confirm the neutrino flux predictions for the Standard Solar Model.
Since the standard solar model was composed using information derived from accelerator experiments, it may be inherent to its design to reflect the neutrino production of the particle accelerators in the coronal clouds around the Sun.
Neutrinos from the Sun results
The coronal energy section suggests that the energy sufficient for the fusion believed to be going on in the core of the Sun may actually be present above the photosphere.
As the coronal cloud is believed to regenerate itself quite readily the vast majority of energetic protons may escape and be readily replaced to continue the fusion. But, this suggests that additional energy may be coming from somewhere else.
The hardness of the proton spectrum may be amplified by the accelerating magnetic fields.
Any sign of variation may not be relevant.
Since the standard solar model was composed using information derived from accelerator experiments, it may be inherent to its design to reflect the neutrino production of the particle accelerators in the coronal clouds around the Sun.
Neutrinos from the Sun discussion
The experimental evidence discovered so far suggests the likelihood that most or all neutrinos may be produced above the photosphere. This may be accomplished in many different ways. In turn, these alternatives allow each endeavor to have impact and potential.
The large factors of 2 1/2 to 6 times the neutrino production rate suggests that much less energy is needed to produce the neutrino level of a quiet Sun.
The large amounts of fusion that has evidenced itself in the section on coronal energy is receiving its energy from somewhere. Probably not from internal sources.
Neutrinos from the Sun conclusions
It may be that the neutrinos that emanate from the solar octant come from above the Sun as part of an interstellar flow that heats the Sun externally.
Neutrinos from the Sun report
Title
Neutrinos from Around the Sun
by --Marshallsumter (discuss • contribs) 04:19, 12 March 2014 (UTC)
Abstract
Experimental evidence has been gathered together to suggest that most and perhaps all neutrinos detected from the solar octant emanate from a shell around the Sun rather than from the core of the Sun. Lines of analysis exist for examining this contention to determine its validity or its shortcomings.
Introduction
Although neutrinos may originate from inside the Sun, it is equally likely and maybe more so that external heating of the Sun by an interstellar electron influx energizes the coronal cloud that in turn creates neutrinos in an apparent relentless heating of the gas cloud that is the Sun. From earlier correlations between the solar cycle and the planets it may be also that the sunspot cycle relates to electron flows from the planets that intensify the neutrino production.
Experiment
The experimentation consists of trying to organize what experimental evidence exists into sections to address the concerns described as necessary to show that neutrinos come from above and around the Sun.
This allows others to alter or improve to determine if the idea of external heating has more merit than gravitational collapse.
The experiments consist of at least five parts:
- energetic protons,
- coronal energy,
- proton escape,
- proton hardness, and
- sign or oscillatory variation.
Results
The organization appears to be complimentary to the idea that external heating drives external fusion. How the influx of electrons brings about the solar cycle and the variations in the neutrino flux is suggested. The outflux of the fast and slow solar wind may be an insufficient loss of energetic protons to reduce or prevent surface or chromosphere fusion processes.
Discussion
Many formula details have not been described to present a complete picture of the heating process and how this electron influx produces the various magnetic and electric manifestations observed.
That each type of neutrino can occur depending on the subatomic particle interactions may be good news for contributors.
Conclusion
A preliminary outline has been suggested of how the heating of the Sun is being accomplished.
Neutrinos from the Sun evaluation
To assess your idea on how to determine where the neutrinos are coming from, including your justification, analysis and discussion, I will provide such an assessment of my example for comparison.
Evaluation
Probably the major shortcoming is the details of the mechanism for solar heating itself and how it spheres the Sun.
Particle accelerators
Polar reversal laboratory
Radiation astronomy empiricisms laboratory
Vega spectrum laboratory
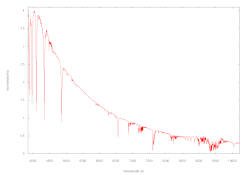
Vega is a nearby star that has one of its poles facing Earth. A number of spectra for this star have been recorded, with at least one in the public domain.
This laboratory is an activity for you to analyze the spectrum of Vega, or any star of your choice that you can find a spectrum for. While it is part of the astronomy course principles of radiation astronomy, it is also independent.
Some suggested entities to consider are emission lines, continua, background, absorption, reflection, or protoplanetary disk.
Spectrum of Vega control group
For creating an analysis of the spectrum of Vega, what would make an acceptable control group? Think about a control group to compare your spectral analysis or your process of creating such an analysis to.
Spectrum of Vega sampling
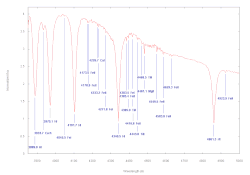
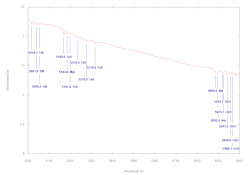
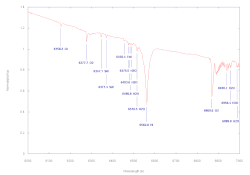
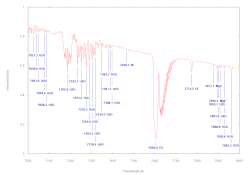
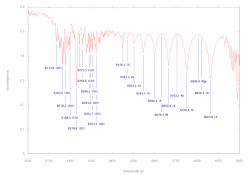
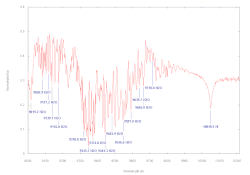
At the top right of this resource is an instrumentally corrected full spectrum of the star Vega.
The second part of the laboratory is to produce or locate an annotated spectrum or set of spectra that indicate which lines come from what elements.
Each of the annotated spectra at right display portions of the spectrum at the top of this resource:
- the wavelength domain from 380.0 nm to 500.0 nm,
- the 500.0-600.0 nm domain,
- the 600.0-700.0 nm domain,
- the 700.0-800.0 nm domain,
- the 800.0-900.0 nm domain, and
- the 900.0-1020.0 nm domain.
Each of these images at right has various element lines labeled: calcium, hydrogen, iron, magnesium, nitrogen, oxygen, silicon, sodium, and titanium.
Molecular lines occur for H2O and O2 which are likely from Earth's atmosphere or the accretion disk around Vega.
The applicable astronomies are
- violet astronomy, blue astronomy, cyan astronomy, and green astronomy,
- green astronomy, yellow astronomy, and red astronomy,
- red astronomy and near infrared astronomy,
- near infrared astronomy,
- near infrared astronomy, and
- near infrared astronomy.
The principal low atomic number elements of interest, whose lines need to be identified are
- hydrogen,
- helium,
- lithium,
- beryllium,
- boron,
- carbon,
- nitrogen,
- oxygen,
- fluorine, and
- neon.
Identification of ionization state should be made where possible.
Spectrum of Vega verification
Element lines and wavelengths can be verified using these resources:
- Alexander Kramida; Yuri Ralchenko; Joseph Reader (September 24, 2012). NIST Atomic Spectra Database, Version 5. Gaithersburg, Maryland, USA: National Institutes of Standards and Technology (NIST). Retrieved 2013-01-20.
- Jean E. Sansonetti; W. C. Martin; S. L. Young (December 9, 2011). Handbook of Basic Atomic Spectroscopic Data. Gaithersburg, Maryland, USA: Physical Measurement Laboratory, NIST. Retrieved 2013-01-24.
The element lines to look for and verify whether present of not are
- hydrogen, all high intensity lines detected and state properly labeled.
- helium, the two major lines at 388.86 and 706.519 nm are possible but may overlap the hydrogen line at 388.90 nm.
- lithium - no lines detected.
- beryllium - no lines detected.
- boron - two strong lines are possible, the 412.1933 nm as a shoulder on H I 410.17 nm line, while the other 448.705 nm overlaps the labeled 448.11 Mg II line.
- carbon, the line at 723.642 nm is possible but unlabeled.
- nitrogen, high intensity lines labeled, but the 868.028 N I is possible as an unlabeled shoulder.
- oxygen - the 777.42 nm O I may be mislabeled and should be 777.194 nm O I which is the stronger line.
- fluorine - the 685.603 nm F I line is possible.
- neon - not detected.
For the heavier elements:
- sodium - the two Na I lines are properly labeled.
- magnesium - all of the lines may be wrong and mislabeled.
- calcium - all high intensity lines present and labeled, the Ca K is a Ca II line.
- titanium - the Ti II line at 439.50 nm is properly labeled as are the others.
- silicon - lines are properly labeled.
- iron - all are labeled properly, because the 501.84 nm line is only Fe II, which differentiates Fe I lines from Fe II lines.
Spectrum of Vega report
Title The element lines of the spectrum of Vega between 388.0 nm and 1020.0 nm.
by Marshallsumter (discuss • contribs) 19:35, 24 March 2014 (UTC)
Abstract
A detailed spectrum of starlight from the star Vega was collected on July 4, 2002, using a commercially available spectrometer. The lines were indexed and labeled as to element and ionization state. This analysis is a subsequent examination of the spectrum for possible misidentification, improper ionization state, and potential red shift or blue shift.
Introduction
Vega is a nearby optical spectral type A0V star at about 25 lyrs. Its polar effective temperature is near 10,000 K, while its equatorial effective temperature is 7,600 K. Vega is a known soft X-ray source. If an X-ray classification of stars is possible, the cloud that became Vega may have any composition initially. Any surface fusion ongoing above the photosphere may be expected to produce some lighter elements. An examination of its spectrum may indicate some of these light elements.
Experiment
The full optical spectrum of Vega between 388.0 nm and 1020.0 nm should reveal the protostar cloud initial composition plus atmospheric fusion products. Its soft X-ray output suggests only lower energy fusion products, if any. Spectral components from about 380.0-500.0 nm, 500.0-600.0 nm, 600.0-700.0 nm, 700.0-800.0 nm, 800.0-900.0 nm, and 900.0-1020.0 nm were examined for proper and complete labeling of peaks.
Results
All high intensity hydrogen lines were detected and together with ionization states properly labeled. Helium lines (two) may be present but overlap the hydrogen lines. No lithium or beryllium was detected. Boron lines may be mislabeled as magnesium lines. The carbon line at 732.642 nm is a possible detection but is unlabeled. The nitrogen high intensity lines are present and labeled, and 868.028 nm N I line is possible as an unlabeled shoulder. Oxygen is present as monatomic although the 777.42 nm O I line may be mislabeled and should be the 777.194 nm O I line which is stronger. The high intensity fluorine line at 685.603 nm F I is possible, appears to be present, but is unlabeled. Neon was not detected.
Of the heavier elements, the alkali and alkali-earth sodium and calcium were detected. The metals titanium and iron are properly labeled and the Fe II line 501.84 nm is present and allows the differentiation between Fe I and Fe II ionization states. Silicon lines are present and properly labeled.
No red shift of blue shift is apparent.
Discussion
The over riding presence of hydrogen suggests that very little atmospheric surface fusion has taken place. This is supported by the possible presence of boron. The presence of carbon, nitrogen, oxygen, and likely fluorine suggests that subsequent lower energy fusion has been consuming, or may have consumed the helium, lithium, and beryllium. Without neon being detected it is likely that the heavier elements are dust and debris captured by Vega or present as dust in the initial cloud before it was energized.
Conclusion
Vega is an optical spectral type A0V star that appears to have very little ongoing atmospheric surface fusion. Its current spectrum suggests only that the original cloud has been energized and contained heavier elements. The high surface temperature suggests that any electron beam heating is primarily directed toward the photosphere. As Vega has a pole towards Earth that may also be in a coronal hole, assessment of its potential X-ray classification is difficult.
Spectrum of Vega evaluation
To assess your spectral analysis, including your justification, analysis and discussion, I will provide such an assessment of my example for comparison.
Evaluation
No evidence is presented for declaring that the magnesium labeled lines are likely boron except comparison with NIST computer generated lines matched with observations. More detailed examination of the lines present, including the possible missing helium may suggest a different evolution for Vega than the one presented. Evidence allowing a choice between alternative evolutions was not presented or discussed.
Standard candles laboratory
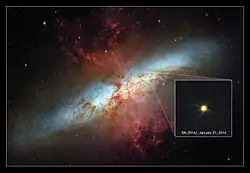
A large number of standard candles have been used over the recent history of stellar and galactic research to estimate distances for sources well beyond those calculated from visual trigonometric parallax.
This laboratory is an activity for you to evaluate a standard candle of your choosing.
While it is part of the astronomy course principles of radiation astronomy, it is also independent.
Some suggested entities to consider are brightness, diameter, tilt, emission lines, absorption lines, periodic variability, mass, time, Euclidean space, Non-Euclidean space, or spacetime.
Standard candles control group
The findings demonstrate a statistically systematic change from the status quo or the control group.
For creating a standard candle, what would make an acceptable control group? Think about a control group to compare your standard candle or your process of creating a standard candle to.
Standard candles experimentation
The star Betelgeuse may still be too far away for visual trigonometric parallax. Standard candles have probably been used to estimate its distance from the Sun. Estimates from visual trigonometric parallax may be available to evaluate the historical accuracy of standard stellar candles.
In 1977 the first direct angular-diameter observations of 119 Tauri were made.[36]
As a spectral type M2.2 Iab information is inferred about Betelgeuse (a type M2.2 Iab) from the occultation measurements of 119 Tauri.[36] The occultations were on January 31, and April 23, 1977.
The spectral type of 119 Tau has been constant since 1940.[36]
In 1977, 119 Tauri (CE Tau) was a spectral type M2.2 Iab, with a spectral range of M2.0-M2.4 Iab-Ib.[36]
In 1977, Betelgeuse was a spectral type M2.0 Iab-, with a range of M1.3-M2.8 Iab-Ib.[36]
For α Sco in 1980 it was M1.1 Iab with a range of M0.7-M1.5 Iab-Ib
As of 2014, 119 Tauri is an M2Iab according to SIMBAD.
As of 2014, Betelgeuse (alf Ori) is an M2Iab according to SIMBAD.
"The spectral type of α Ori varies roughly with the 5.8 yr period and epoch [...] for brightness, radial velocity, and possible angular-diameter variations. Recently, α Ori has shown the latest spectral type between 1973 and 1975 and again in 1980 January-February with a spectral type of M2.8 Iab-. Its spectral type was about M1.5 around 1969-1971 and again around 1977-1978. By 1982 or 1983, α Ori should again have a spectral type of about M1.5."[36]
In 1977, apparently α Scorpii (Antares) was an M2.2 Iab, but in 2014 it is an M1.5Iab-b.
The standard candle being used in 1977 for spectral region K5-M4 is the CN (cyanide) index from the CN absorption in selected bands.[36]
The apparent magnitude needed for calculating an object's distance in pc is obtained using a photospheric magnitude received for the 1.04 µm flux peak, I(104) in early-M stars.[36]
Near-infrared "photometry on the narrow-band eight-color system [...] has been obtained for these stars. The mean [CN] indices and spectral types derived from photometry of the three supergiants are"[36]
- 119 Tau, CN index = 18 ± 2, I(104) mag = +0.84 ± 0.03, MV = -5.2 (-4.8 to -5.6), distance = 417 pc,
- α Ori, CN index = 18 ± 3, I(104) mag = -2.68 ± 0.03, MV = -5.2, (-4.5 to -5.8), distance = 96 pc, and
- α Sco, CN index = 19 ± 3, I(104) mag = -2.28 ± 0.02, MV = -5.5, (-4.5 to -5.9), distance = 107 pc.
"The distance to α Ori is about half the value, 200 pc, that is almost universally used in the literature."[36]
"The direct evidence for a distance of 200 pc [to Betelgeuse] is a trigonometric parallax of 0.005, which is 10 times smaller than the expected error of measurement [0.005 ± 0.05 mas]."[36]
Standard candles results
In 1977 using the absorption spectrum of cyanide believed to be applicable for the spectral region K5-M4 to produce a CN index and the relationship between apparent magnitude and absolute magnitude, a distance of 96 pc was estimated for Betelgeuse. Parallax measurements at that time estimated a distance of 200 pc, but the error was 10 times greater than the value derived.[36]
A parallax measurement by the satellite Hipparcos indicated a distance of 197 ± 45 pc published in 2008.
While post 1980 adjustments were made to increase the estimated distance of Betelgeuse, the initial discrepancy is quite large, at least a factor of 2 using a standard candle.
Standard candles discussion
Estimates made using standard candles rather than some type of direct measurement are expected to be inaccurate.
In 1977, the distance to Betelgeuse estimated by various standard candles suggested 200 pc, "almost universally used in the literature."[36]
Adjustments after 1980 to the standard candle used to estimate the absolute magnitude of Betelgeuse and the 96 pc distance tended toward the universally accepted value.
The ESA's Gaia spacecraft is expected to start collecting data in May 2014 on approximately 109 Milky Way objects. This data will be used to determine their parallax and relative motions. Betelgeuse is a likely target.
Standard candles are hoped to achieve distances within an order of magnitude of reality. It may take another 50 years to produce a device beyond the technology of Gaia to determine distances directly to galaxies beyond the Milky Way.
Standard candles conclusion
The analysis of an attempt at a more precise distance to Betelgeuse which in 1977 was beyond direct measurement by trigonometric parallax demonstrates both the strengths and weaknesses of indirect measurements or estimates. Astronomical objects may be sufficiently novel, one to another, to make standard candles at best only good to an order of magnitude.
Standard candles report
Title
A standard candle distance to Betelgeuse
by Marshallsumter (discuss • contribs) 19:57, 27 March 2014 (UTC)
Abstract
The standard candle of the absorption by cyanide (CN) to produce a CN index applicable to the spectral region K5-M4 was analyzed for the star Betelgeuse. The underestimate of the distance to Betelgeuse demonstrates both the value and limitations of standard candles.
Introduction
In 1977 the direct measurement of the distance to Betelgeuse was lacking. To fill this gap, an accepted standard candle was used to estimate the absolute magnitude of Betelgeuse. Once estimated, an apparent magnitude obtained in the infrared provided an estimate of the distance.
Experiment
The experimental effort was to locate and analyze the test of a standard candle for the distance to Betelgeuse.
In 1977 using the absorption spectrum of cyanide believed to be applicable for the spectral region K5-M4 to produce a CN index and the relationship between apparent magnitude and absolute magnitude, a distance of 96 pc was estimated for Betelgeuse.
Results
The estimate did not conform to a universally accepted distance to Betelgeuse. The standard candle with some adjustments seems to be well founded in a qualitative approach to distance estimates currently beyond direct measurement.
Discussion
Any effort to make a standard candle more accurate than an order of magnitude as a replacement for direct measurement is likely to be inaccurate.
Conclusion
Standard candles fill in a necessary gap between techniques of direct distance measurement and back of the envelope guesses.
Standard candles evaluation
To assess your standard candle, including your justification, analysis and discussion, I will provide such an assessment of my example for comparison.
Evaluation
Using only one standard candle is anecdotal. At least five separate standard candles, especially four others that were much closer to the universally accepted distance for Betelgeuse should have been included. This would have presented a more statistically sound treatment of standard candles.
Vertical precession laboratory
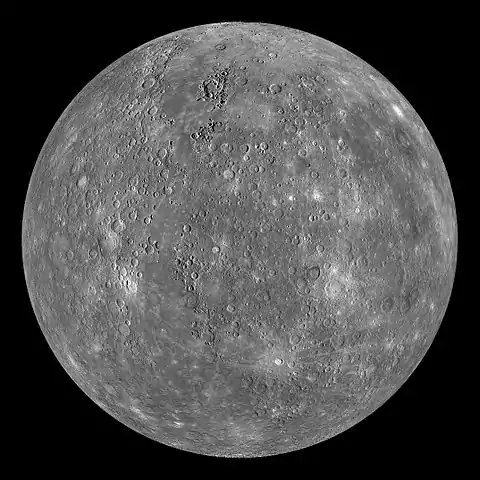
Precession of orbital axes in the plane of the ecliptic is a common occurrence and easily described using tidal effects of other planets of massive bodies in the ecliptic.
This laboratory is an activity for you to evaluate possible sources for the vertical precession of a planetary orbit.
A vertical precession describes precession of orbital axes out of and back across the ecliptic. These are not so easily explained by the presence of other massive bodies in the plane of the ecliptic.
Some suggested entities to consider for calculating and evaluating vertical precession of a planet of your choice are electric fields, tidal effects, mass, time, Euclidean space, Non-Euclidean space, or spacetime.
Vertical precession control group
For creating a vertical precession force system, what would make an acceptable control group? Think about a control group to compare your vertical precession forces or your process of creating vertical precession to.
Orbital inclination

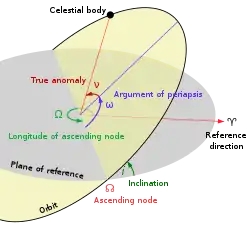
Def. the "angle of intersection of a reference plane"[37] is called an inclination.
"The orbital inclination [(i) of Mercury] varies between 5° and 10° with a 106 yr period with smaller amplitude variations with a period of about 105 yr."[38]
"Inclination of [Mercury's] orbit to [the] ecliptic [is] 5.15° [on or about July 20, 2010.]"[39]
"Mercury Mean Orbital Elements (J2000) [...] Orbital inclination (deg) 7.00487 [...] Reference Date : 12:00 UT 1 Jan 2000 (JD 2451545.0) [...] Last Updated: 01 July 2013, DRW [David R. Williams]."[40]
"The next largest [orbital inclination as of September 13, 2006,] is Mercury which orbits 7° from Earth."[41]
"Mercury [...] Inclination [is] 7.00559432 degrees [as of March 20, 2014]."[42]
The graphs at the top show the initial planetary orbital elements for the planet Mercury taken from the Development Ephemeris of JPL, DE245 (cf. Standish 1990) in the left portion marked (e) and the final integration results on the right (m).[43]
The projected time spans from the integrations suggest that conditions within the solar system for the recorded data set are very stable.
Vertical precession report
Title
The vertical precession of the orbit of Mercury
by Marshallsumter (discuss • contribs) 00:54, 1 April 2014 (UTC)
Abstract
Using the available ephemeris for the orbital elements of the planet Mercury, computer calculations appear to have demonstrated that whatever forces are at work in the solar system are producing a reasonably stable system. Data is only available for the orbit of Mercury from several hundred years of observations.
Introduction
Some of the forces present in the solar system during the course of data collection include gravity, electron influx from the interstellar medium, and outflux of neutrals and charged particles from the Sun (the solar wind).
A system such as the solar system may become "unstable when a close encounter occurs somewhere in the system, starting from a certain initial configuration. A system is defined as experiencing a close encounter when two bodies approach one another within an area of the larger Hill radius."[43]
Experiment
The experiment has consisted of locating data on the vertical precession of Mercury's orbit with respect to the ecliptic. In the choice of planet, at least one computer study has been performed using data available to provide integrations over time to test the overall planetary system for unstable conditions.
Results
These very long-term numerical integrations suggest that the present planetary configuration and interaction appears stable.
Discussion
The computer integrations for available data on the planet Mercury are limited to an apparently very stable period in the recorded history of the solar system. Significant changes in charge particle influx or outflux apparently have not occurred. Nor have any significant interstellar bodies of large size entered or passed through the solar system. The same may be said for large clouds of any kind. Supernovae have been observed at distances that have precipitated little of no effect on the solar system.
Conclusion
Although forces persist within the solar system that may under certain circumstance produce one or more close encounters, none apparently have occurred at least within the last several hundred years. The combination of forces within the solar system appear to be sufficiently balanced to allow the vertical precession of Mercury's orbit without specific delineation of their effects on this precession.
Vertical precession evaluation
To assess your vertical precession force system, including your justification, analysis and discussion, I will provide such an assessment of my examples for comparison.
Evaluation
A specific interaction of forces within the present solar system has not been performed to test any hypothesis. Letting the apparent stability of the solar system be described by a computer integration may be little more than sticking one's head into the sand like an ostrich.
X-ray classification of a star laboratory
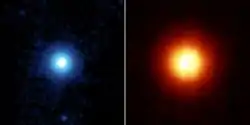
This laboratory is an activity for you to classify a star of your choice using its X-ray characteristics. While it is part of the astronomy course principles of radiation astronomy, it is also independent.
Some suggested classification entities to consider are wavelength range, periodicity, spectrum, mass, binarity, Euclidean space, Non-Euclidean space, or spacetime.
X-ray classification of a star control group
The findings demonstrate a statistically systematic change from the status quo or the control group.
For creating an X-ray classification of your star, what would make an acceptable control group? Think about a control group to compare your classification of your star or your process of classifying it too.
One likely control group is the visual classification of stars.
Candidate stars
According to SIMBAD, Vega (alpha Lyrae) (delta Sct type variable) is an X-ray source in the first Einstein catalog (1E) and an ultraviolet source from the CEL, EUVE, and TD1 catalogs. It has not been detected as a gamma-ray source.
At one time (1932), Vega was listed as a double star.[44] Also in 1963, Vega is listed as a visual double star.[45] Again in 1983, it was listed as a double star.[46] This was repeated in 1994.[47] It is still listed as a double star in 1996.[48] The update in 2001 also lists it.[49] The star with Vega is 56.41 arcsec away and is designated as BD+38 3238D of unknown spectral class. That these two stars are undifferentiated between double star or binary star for some 70 years at only 25 lyrs away is remarkable.
The images at the top of the resource has a 24-micron image in blue on the left and a 70-micron infrared image on the right. "The [debris] disc extends to at least 815 astronomical units."[50] "The images are 3 arcminutes on each side."[50] BD+38 3238D is unspecified or unlocated within the infrared images but should be within the debris disc. According to SIMBAD there are ten objects within one arcminute of Vega including BD+38 3238D, a submillimeter source (JCMTSE J183656.4+384709), and eight infrared sources: [ MHW2003] 1-8.
X-ray analysis
The X-ray "counts observed from [...] Vega in the HRI are very likely to be due entirely to UV contamination from the photospheric emission, and hence the X-ray luminosities for [...] Vega [...] should be replaced by upper limits at least one order of magnitude lower, i.e., log LX, Vega < 26.6 [...] Thus the HRI observation of Vega is completely consistent with the upper limit obtained in the IPC, and the only remaining inconsistency concerning the X-ray emission from Vega is the rocket experiment by Topka et al. (1979), who report a detection of Vega in a 5 s pointing yielding 7 counts; however, in our opinion these authors do not convincingly rule out the possibility of UV contamination. Note in this context that the IPC's used for Topka et al.s' (1979) rocket flight and for the Einstein Observatory were not identical."[51]
"Many types of main sequence stars emit in the X-ray portion of the spectra. In massive stars, strong stellar winds ripping through the extended atmosphere of the star create X-ray photons. On lower mass stars, magnetic fields twisting through the photosphere heat it sufficiently to produce X-rays. But between these two mechanisms, in the late B to mid A classes of stars, neither of these mechanisms should be sufficient to produce X-rays. Yet when X-ray telescopes examined these stars, many were found to produce X-rays just the same."[52]
"The first exploration into the X-ray emission of this class of stars was the Einstein Observatory, launched in 1978 and deorbited in 1982. While the telescoped confirmed that these B and A stars had significantly less X-ray emission overall, seven of the 35 A type stars still had some emission. Four of these were confirmed as being in binary systems in which the secondary stars could be the source of the emission, leaving three of seven with unaccounted for X-rays."[52]
"The German ROSAT satellite found similar results, detecting 232 X-ray stars in this range. Studies explored connections with irregularities in the spectra of these stars and rotational velocities, but found no correlation with either. The suspicion was that these stars simply hid undetected, lower mass companions."[52]
Either "the main star truly is the source, or there are even more elusive, sub-arcsecond binaries skewing the data."[52]
On July 27, 1977, at 05:41:48.1 UTC, an Aerobee 350 or boosted Black Brant launched from White Sands Missile Range using Vega as a reference by its star tracker to update its position while maneuvering between X-ray targets automatically observed Vega with its X-ray telescope for 4.8 s.[53]
The quantity of detected photons (7) in the band 0.2-0.80 keV corresponds to an X-ray luminosity LX ≈ 3 x 1028 erg s-1.[53]
"The ANS 3 σ upper limit for Vega (2.5 x 1028 ergs s-1) is only slightly lower than our flux measurement."[53]
"Because the X-ray [luminosity] of Vega [is] much closer to that of the Sun than to the typical galactic X-ray sources which have been detected to date, it is natural to consider processes analogous to solar coronal activity as the explanation for the X-ray activity."[53]
"Vega is thought to be a solitary star, and therefore noncoronal X-ray-producing mechanisms seem to be excluded".[53]
"Vega is the first solitary main-sequence star beyond the Sun known to be an X-ray emitter".[53]
Vega's "computed X-ray surface luminosity [...] is comparable to that of the quiet Sun [...] Note, however, that because of our very short exposure, the average level of coronal emission may vary significantly from our single measurement."[53]
"Using estimates of the stellar [radius] derived from stelar structure calculations, we obtain [a] surface X-ray [luminosity] of ~6.4 x 104 ergs cm-2 s-1 for Vega [that falls] within the range of solar coronal X-ray emission, which can vary between ~8 x 103 ergs cm-2 s-1 in coronal holes and ~3 x 106 ergs cm-2 s-1 in active regions".[53]
Magnetic "field activity, leading to coronal heating, may account for Vega's X-ray emission because of inhomogeneous distribution of surface magnetic flux and associated coronal activity."[53]
That Vega is regarded as an X-ray source rests on one 4.8 s star-tracking observation by one sounding rocket flight carrying an X-ray detector flown on many flights that yields trustworthy results.
"Vega is a pole-on, highly oblate, rapid rotator [...] the star exhibits extreme limb darkening and a large decrement in effective temperature from pole to equator. [...] the best fittingmodel (Teff pole=10150 K, Teff eq = 7900 K, θ = 3.329 mas) has the pole inclined 5° to line of sight and rotates at 91% of the angular speed of break-up, resulting in a temperature drop of 2250 K from center to limb. [...] the total luminosity [...] is emitted in a highly non-homogeneous manner with five times more UV flux being emitted from the pole as is emitted in the equatorial plane, while the visible through near-IR flux is some 70% greater at the pole than that of the equatorial plane and 54% greater than that expected from a slow or non-rotating A0 V star."[54]
A 'polar coronal hole' is a coronal hole that occurs above one or both rotational poles of a star that has a coronal cloud around it.
"The radiant emission from coronal holes is greatly diminished relative to other coronal regions".[55]
The "emission is proportional to the integral of the square of the electron density along the line of sight [...] Data of this type are therefore heavily influenced by regions of high density along the line of sight--the low corona for disk observations, and denser structures surrounding coronal holes for limb observations."[55]
An "analysis of the northern polar region during the period 1973 June 29 to July 13 [...] can be summarized as follows. The boundary of the hole is essentially axisymmetrc about the polar axis and is nearly radial from 3 to 6 R⊙. The boundary at these heights is located at 25° ± 5° latitude, although it is of much smaller extent (boundary ~65° latitude) as observed near the solar surface with the American Science and Engineering (AS&E) X-ray experiment on Skylab [...] the increase of the polar hole's cross sectional area from the surface to 3 R⊙ is approximately 7 times greater than for a purely radial boundary."[55]
For α Lyr, log FX/FV = -6.79 (variable X-ray source), log LX 27.6 erg s-1 (variable X-ray source).[56] Upper limits were log FX/FV = -7.4 and log LX 27.0 erg s-1.[56]
A coronal cloud is not a diffuse, homogeneous hot atmosphere, but one or more strongly structured topologically closed features dominated by magnetic confinement.
Magnetic field of Vega
An extensive convection zone is not required, and any star with magnetic field strengths and geometry similar to the Sun's will possess a corona.[53]
Magnetic fields on the order of ~30 gauss have been reported for Vega (~1 gauss for the Sun), so perhaps these substantially higher average field strengths compensate for the expected reduced convective activity, resulting in surface X-ray luminosities comparable to the quiet Sun.[53]
Using spectropolarimetry, a magnetic field has been detected on the surface of Vega by a team of astronomers at the Observatoire du Pic du Midi.[57] They "report the detection of a magnetic field on Vega and argue that Vega is probably the first member of a new class of yet undetected magnetic A-type stars."[57]
The "polarization [is] a Zeeman signature [that] leads to a value of [Bl =] -0.6 ± 0.3 G for the disk-averaged line-of-sight component of the surface magnetic field."[57]
"The strength of Vega magnetic field is about 50 micro-tesla, which is close to that of the mean field on Earth and on the Sun."[58]
Rotational velocity of Vega
"Vega rotates in less than a day, while the Sun's rotation period is 27 days."[58]
Infrared analysis
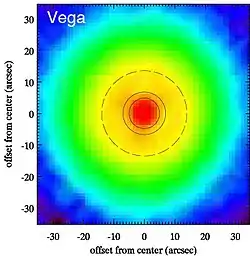
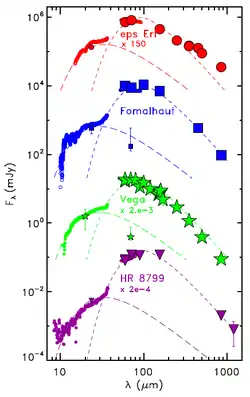
"The infrared excesses are well modeled by two components, a warm belt close to the star, and a cooler belt farther out. The clear separation of the belts could be explained by the presence of planets clearing the gap."[59]
The graph at left shows the clear separation of infrared belts for Vega. This separation may "be explained by the presence of planets clearing the gap."[59]
Sun as an X-ray source
The photosphere of the Sun does not emit X-rays. The chromosphere and the transition region either emit ultraviolet, extreme ultraviolet, or X-rays (most likely soft X-rays). The coronal clouds around the Sun emit X-rays and sometimes gamma-rays, neutrinos, neutrals, protons, positrons, and electrons, among other solar cosmic-rays.
X-ray classification of a star report
Title
An X-ray classification of the star Vega (α Lyrae)
by Marshallsumter (discuss • contribs) 02:16, 7 April 2014 (UTC)
Abstract
The X-ray characteristics of the Sun have been studied since the 1940s. The may be considered an X-ray variable star as its intensity corresponds to the sunspot cycle. This cycle may have its origins in the mechanisms that heat the photosphere and the coronal clouds around the Sun. An analysis of the X-ray characteristics of Vega suggest that it is a Sun-like X-ray star.
Introduction
It has taken a number of decades to observe and describe the X-ray properties of Vega. Associated with these properties are a magnetic field comparable to the Sun and the possibility of a coronal hole over the pole facing Earth.
Experiment
By scanning the available literature to ascertain the X-ray properties of Vega, several apparent conclusions may be drawn. The X-ray output of Vega falls between that of the Sun. The X-ray output of the Sun minimizes during the solar cycle quiet period and maximizes at the sunspot maximum.
Vega is considered an X-ray variable star with a corona and an X-ray output comparable to the Sun.
Results
Vega is considered to be Sun-like in its X-ray output and variability. The peak of X-ray output may not be directly observable as the star has a pole facing Earth.
Discussion
The photosphere of Vega has a diameter five times that of the Sun. Its apparent stellar system has similarities to the solar system but is scaled as approximately four times larger. Even with a pole temperature above 10,000 K Vega produces no detectable X-ray output from its photosphere, just like the Sun at 5,778 K. Vega is a visual spectral type A0V star which means it is transparent. The Sun is not considered transparent at visual wavelengths.
The physical size of the photosphere of Vega and its transparency may reflect more the cloud it formed from than anything else.
The above surface fusion that is occurring on the Sun may not be happening above the photosphere of Vega as no flares or gamma rays have been detected.
Conclusion
Vega is a Sun-like X-ray source.
X-ray classification of a star evaluation
Even the visual classification of stars uses more than just visual characteristics general to the star. It uses spectral lines a specific elements, size, shape, metallicity, and special elemental compositions. Classifying Vega solely on the basis of its apparent X-ray characteristics and leaving the other properties to its cloud of origin seems short-sighted.
X-ray trigonometric parallax laboratory
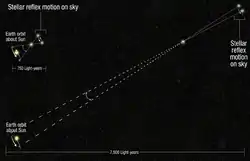
This laboratory is an activity for you to perform X-ray trigonometric parallax on a star, or other nearby X-ray source of your choice using its X-ray characteristics. While it is part of the astronomy course principles of radiation astronomy, it is also independent.
Some suggested trigonometric entities to consider are wavelength range, periodicity, spectrum, background X-ray sources, mass, binarity, Euclidean space, Non-Euclidean space, or spacetime.
More importantly, there are your X-ray trigonometric entities. Search SIMBAD for your star, or nearby X-ray source, to be sure it has been detected as an X-ray source.
You may choose to define your X-ray trigonometric parallax entities or use those already available.
Usually, research follows someone else's ideas of how to do something. But, in this laboratory you can create these too.
X-ray trigonometric parallax control group
The findings demonstrate a statistically systematic change from the status quo or the control group.
For determining an X-ray trigonometric parallax of your star or other nearby X-ray source, what would make an acceptable control group? Think about a control group to compare your determination of your star or your process of determining its parallax to.
X-ray trigonometric parallax sampling
Trigonometric parallax may be somewhat wavelength dependent. Usually, it depends on resolution and using a periodic set of measurements where the diameter of the period is large enough to allow resolution. To attempt X-ray trigonometric parallax, a candidate star that is within resolution of at least one currently available or formerly available X-ray satellite is needed. If none qualify for even the closest star, then a calculation that is time based may work or a calculation using a greater periodic set is needed.
For the effort to succeed a collection of three to five very distant, unmoving X-ray sources must be available to determine the target's relative movement.
Since the idea of using X-ray astronomy satellites is novel and perhaps a bit premature, finding a relatively nearby source where the experiment may be performed may be itself a time-consuming task too soon for a course.
To test even this hypothesis, I choose Proxima Centauri.
According to SIMBAD, V645 Cen (Proxima Centauri) is an X-ray source in the catalogs: 1E, 2E, 1ES, RE, RX, 1RXS, and [FS2003]. Catalogs 1E through 1ES are the Einstein satellite observations. Catalogs RE through 1RXS are the ROSAT satellite observations. Catalog [FS2003] is a systematic search for variability among ROSAT All-Sky Survey X-ray sources by B. Fuhrmeister and J. H. M. M. Schmitt in an article published in Astronomy and Astrophysics.
The X-ray resolutions of these two satellites are
- High Energy Astrophysics Observatory 2 (Einstein X-ray Observatory) - "a spatial resolution of ~1´."[60] and
- ROSAT - "~ 2 arcsec spatial resolution (FWHM)".[61]
The visual astronomy parallaxes (mas) on Proxima Centauri are 774.25 ± 2.08, according to SIMBAD. As the resolution of neither Earth-orbit satellite is within the parallax range it is unlikely that any sets of X-ray observations from these two satellites can resolve parallax movement by Proxima Centauri.
Perhaps one of the current X-ray satellites has sufficient resolution:
- AGILE - not stated so far,
- Chandra X-ray Observatory (Advanced X-ray Astrophysics Facility, (AXAF)) - Spatial resolution < 1 arcsec, HRC-I ~ 0.5 arcsec spatial resolution,
- Fermi Gamma-ray Space Telescope - not stated so far,
- INTEGRAL - spatial resolution 3´,
- MAXI - not stated so far,
- NuSTAR (Nuclear Spectroscopic Telescope Array Mission) - not stated so far,
- Suzaku (Astro-E2) - angular resolution of ~2´ (HPD); all gold-coated,
- Swift X-ray Telescope (XRT) - ~5 arcsec position accuracy, and
- X-ray Multi-Mirror Mission (XMM-Newton) - Spatial resolution 6" FWHM. None of these can perform trigonometric parallax at present, although Chandra may be able to estimate the parallax.
X-ray trigonometric parallax verification
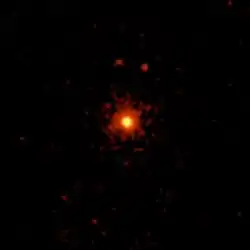
"Chandra and XMM-Newton observations of the red dwarf star Proxima Centauri have shown that its surface is in a state of turmoil. Flares, or explosive outbursts, occur almost continually. This behavior can be traced to Proxima Centauri's low mass, about a tenth that of the Sun. In the cores of low mass stars, nuclear fusion reactions that convert hydrogen to helium proceed very slowly, and create a turbulent, convective motion throughout their interiors. This motion stores up magnetic energy which is often released explosively in the star's upper atmosphere where it produces flares in X-rays and other forms of light."[62]
"The same process produces X-rays on the Sun, but the magnetic energy is released in a less explosive manner through heating loops of gas, with occasional flares. The difference is due to the size of the convection zone, which in a more massive star such as the Sun, is smaller and closer to its surface."[62]
"Red dwarfs are the most common type of star. They have masses between about 8% and 50% of the mass of the Sun. Though they are much dimmer than the Sun, they will shine for much longer - trillions of years in the case of Proxima Centauri, compared to the estimated 10 billion-year lifetime of the Sun."[62]
"X-rays from Proxima Centauri are consistent with a point-like source. The extended X-ray glow is an instrumental effect. The nature of the two dots above the image is unknown - they could be background sources."[62]
"Image is 1.5 arcmin across."[62]
X-ray trigonometric parallax report
Title
Assessment of Performing X-ray Trigonometric Parallax on Proxima Centauri
by --Marshallsumter (discuss • contribs) 18:30, 18 April 2014 (UTC)
Abstract
Many and almost 50% of all X-ray sources have no visual counterpart. These sources may be nearby clouds, hidden stars, or others. Being able to perform X-ray trigonometric parallax would go a long way to determining where and what these sources are. Assessment of the possibility to perform X-ray trigonometric parallax on the nearest X-ray star after the Sun has been performed to determine the apparent state of the art.
Introduction
Of the many X-ray sources in various databases such as SIMBAD, few sources have visual counterparts. Fewer yet have any distance measurements available. With so many stand-alone X-ray sources, it would be worthwhile to assess current X-ray observatory capability for trigonometric parallax.
Experiment
A sampling of X-ray observatories past and current that may have or be capable of performing X-ray trigonometric parallax on Proxima Centauri has been assessed.
Results
At present it appears that no X-ray observatory can or has performed X-ray trigonometric parallax on Proxima Centauri. The Chandra X-ray Observatory may be able to yield a range but has only perceived Proxima Centauri as a point source. Within a 20 arcminute radius of Proxima Centauri there are four known X-ray sources that may be far enough away to be used as parallax immobile X-ray sources, according to SIMBAD.
Discussion
Although an assessment of time-based observations over a multi-year time-frame may show that X-ray trigonometric parallax of a selected target is possible, this may be more time-consuming than productive at this point. An expanded orbit approach using say the Chandra X-ray Observatory at its orbital extremes while at semi-annual Earth orbit extremes is unlikely to increase the resolution significantly.
Conclusion
Current and former X-ray observatory satellites have been in orbit around the Earth since the seventies (1970s) yet it forty years of research apparently not one of these technologies has been developed to permit X-ray trigonometric parallax of any object outside the solar system.
X-ray trigonometric parallax evaluation
To assess your X-ray trigonometric parallax calculations of the star of your choice, including your justification, analysis, results, and discussion, I will provide such an assessment of my example for comparison.
Evaluation
The time necessary to assess other X-ray sources such as Jupiter has not been applied. The Sun could have been used to assess the capability of the technology with the GOES satellites.
Hypotheses
- These astronomy laboratories benefit more from student projects recorded as subpages of their registered user pages.
See also
- Activities/Astronomy
- Problems/Astronomy
- Intergalactic medium
- Meteorites
- Sun
References
- ↑ laboratory. San Francisco, California: Wikimedia Foundation, Inc. September 21, 2013. Retrieved 2013-09-30.
- ↑ P Sommers; S Westerhoff (May 12, 2009). "Cosmic ray astronomy". New Journal of Physics 11 (5): 055004. doi:10.1088/1367-2630/11/5/055004. http://iopscience.iop.org/1367-2630/11/5/055004. Retrieved 2012-03-28.
- ↑ M.K. Khatri; P.R. Poudel; A.K. Gautam. Principles of Physics 2010. Kathmandu: Ayam Publication. pp. 170, 171. ISBN 9789937903844.
- ↑ flux. San Francisco, California: Wikimedia Foundation, Inc. January 24, 2014. Retrieved 2014-01-25.
- ↑ R. Byron Bird; Warren E. Stewart; Edwin N. Lightfoot (2007). Transport Phenomena. John Wiley & Sons. p. 905. ISBN 0470115394.
- ↑ Steve Cole; Jia-Rui C. Cook; Alan Buis (December 2011). NASA's Voyager Hits New Region at Solar System Edge. Washington, DC: NASA. Retrieved 2012-02-09.
- ↑ Brooke Boen (November 8, 2013). NGC 6946: The 'Fireworks Galaxy'. Retrieved 2014-02-08.
- 1 2 Guillermo A. Blanc; Tim Weinzirl; Mimi Song; Amanda Heiderman; Karl Gebhardt; Shardha Jogee; Neal J. Evans II; Remco C. E. van den Bosch et al. (May 2013). "The VIRUS-P Exploration of Nearby Galaxies (VENGA): Survey, Design, Data Processing, and Spectral Analysis Methods". The Astronomical Journal 145 (5): 138. http://iopscience.iop.org/1538-3881/145/5/138/. Retrieved 2014-02-08.
- 1 2 3 4 G. de Vaucouleurs (September 15, 1978). "The extragalactic distance scale. IV - Distances of nearest groups and field galaxies from secondary indicators". The Astrophysical Journal 224 (09): 710-7. http://adsabs.harvard.edu/abs/1978ApJ...224..710D. Retrieved 2014-02-08.
- ↑ Gérard de Vaucouleurs (1994). Global Physical Parameters of Galaxies. http://www.stsci.edu/institute/conference/galaxy-morphology/devaucouleurs.ps. Retrieved 2008-01-02.
- 1 2 A. Evans (January 6, 2002). Hubble Interacting Galaxy UGC 9618. Baltimore, Maryland USA: HubbleSite Newscenter. Retrieved 2014-02-15.
- ↑ Mercury Fact Sheet. NASA Goddard Space Flight Center. November 30, 2007. Retrieved 2008-05-28.
- ↑ Chapman, A. (1985), Transactions of the Historical Society of Lancashire and Cheshire Volume 135 pp 1–14
- ↑ Eric G. Forbes et al. (1995), Correspondence of John Flamsteed Volume 1, Institute of Physics Publishing, p. 624-627
- ↑ Wayne Orchiston 1994, James Cook and the 1769 Transit OF Mercury, Carter Observatory ISSN 1173–7263 http://www.transitofvenus.co.nz/docs/CarterObservatoryInfo3.doc
- ↑ 1960 Transit of Mercury. HM Nautical Almanac Office. UK Hydrographic Office. November 5, 2007. Retrieved May 30, 2012.
- ↑ 1970 Transit of Mercury. HM Nautical Almanac Office. UK Hydrographic Office. November 5, 2007. Retrieved May 30, 2012.
- ↑ 1973 Transit of Mercury. HM Nautical Almanac Office. UK Hydrographic Office. November 5, 2007. Retrieved May 30, 2012.
- ↑ 1986 Transit of Mercury. HM Nautical Almanac Office. UK Hydrographic Office. November 5, 2007. Retrieved May 30, 2012.
- ↑ 1993 Transit of Mercury. HM Nautical Almanac Office. UK Hydrographic Office. November 5, 2007. Retrieved May 30, 2012.
- ↑ 1999 Transit of Mercury. HM Nautical Almanac Office. UK Hydrographic Office. November 5, 2007. Retrieved May 30, 2012.
- ↑ 2003 Transit of Mercury. HM Nautical Almanac Office. UK Hydrographic Office. November 5, 2007. Retrieved May 30, 2012.
- ↑ 2006 Transit of Mercury. HM Nautical Almanac Office. UK Hydrographic Office. November 5, 2007. Retrieved May 30, 2012.
- 1 2 3 Maurice Dubin; Robert K. Soberman (April 1996). "Resolution of the Solar Neutrino Anomaly". arXiv: 1-8. http://arxiv.org/abs/astro-ph/9604074. Retrieved 2012-11-11.
- ↑ A. Bellerive, Review of solar neutrino experiments. Int.J.Mod.Phys. A19 (2004) 1167-1179
- ↑ K.D. Abhyankar. "A Survey of the Solar Atmospheric Models 1977". Bull. Astr. Soc. India 5: 40–44. http://prints.iiap.res.in/handle/2248/510.
- 1 2 3 4 5 6 7 J. N. Bahcall; G. B. Field; W. H. Press (September 1, 1987). "Is solar neutrino capture rate correlated with sunspot number?". The Astrophysical Journal 320 (9): L69-73. doi:10.1086/184978. http://articles.adsabs.harvard.edu//full/1987ApJ...320L..69B/L000069.000.html. Retrieved 2013-07-07.
- 1 2 John N. Bahcall (April 28, 2004). Solving the Mystery of the Missing Neutrinos. Nobel Media AB. Retrieved 2014-03-08.
- ↑ Eberhard Klempt; Chris Batty; Jean-Marc Richard (July 2005). "The antinucleon-nucleon interaction at low energy: annihilation dynamics". Physics Reports 413 (4-5): 197-317. doi:10.1016/j.physrep.2005.03.002. http://adsabs.harvard.edu/abs/2005PhR...413..197K. Retrieved 2014-03-09.
- ↑ Eli Waxman; John Bahcall (December 14, 1998). "High energy neutrinos from astrophysical sources: An upper bound". Physical Review D 59 (2): e023002. doi:10.1103/PhysRevD.59.023002. http://prd.aps.org/abstract/PRD/v59/i2/e023002. Retrieved 2014-03-09.
- 1 2 3 4 5 6 K. Kodama; N. Ushida1; C. Andreopoulos; N. Saoulidou; G. Tzanakos; P. Yager; B. Baller; D. Boehnlein et al. (April 12, 2001). "Observation of tau neutrino interactions". Physics Letters B 504 (3): 218-24. http://www.sciencedirect.com/science/article/pii/S0370269301003070. Retrieved 2014-03-10.
- ↑ Fireman EL; Damico J; Defelice J (March 1975). Solar-wind tritium limit and nuclear processes in the solar atmosphere, In: Lunar Science Conference Proceedings 6th Houston TX. 2. New York: Pergamon Press, Inc.. pp. 1811–21. http://adsabs.harvard.edu/abs/1975LPSC....6.1811F. Retrieved 2014-03-11.
- ↑ Fishman GJ; Harmon BA; Gregory JC; Pamell TA; Peters P; Phillips GW; King SE; August RA et al. (February 19991). "Observation of 7Be on the surface of LDEF spacecraft". Nature 349 (6311): 678-80. doi:10.1038/349678a0.
- ↑ Krčmar, M.; Krečak, Z.; LjubičiĆ, A.; Stipčević, M.; Bradley, D. A. (December 2001). "Search for solar axions using 7Li". Physical Review D (Particles and Fields) 64 (11): 115016-9. doi:10.1103/PhysRevD.64.115016. http://adsabs.harvard.edu/abs/2001PhRvD..64k5016K. Retrieved 2014-03-11.
- 1 2 Fred Espenak (September 8, 2005). Images of the Sun taken by the Transition Region and Coronal Explorer. Palo Alto, California USA: Stanford-Lockheed Institute for Space Research and NASA Small Explorer program. Retrieved 2014-03-11.
- 1 2 3 4 5 6 7 8 9 10 11 12 13 Nathaniel M. White (December 1, 1980). "The Occultation of 119 Tauri and the Effective Temperatures of Three M Supergiants". The Astrophysical Journal 242 (12): 646-56. doi:10.1086/158501. http://articles.adsabs.harvard.edu/full/1980ApJ...242..646W. Retrieved 2014-03-26.
- ↑ inclination. San Francisco, California: Wikimedia Foundation, Inc. December 14, 2012. Retrieved 2013-02-01.
- ↑ Peale, S. J. (June 1974). "Possible histories of the obliquity of Mercury". Astronomical Journal 79 (6): 722-44. doi:10.1086/111604.
- ↑ A. Odman (July 20, 2010). Inner Planets part A Mercury and the Moon. Portland, Oregon USA: Portland Community College. Retrieved 2014-03-31.
- ↑ David R. Williams (January 1, 2000). Mercury Fact Sheet. Greenbelt, MD, USA: NASA Goddard Space Flight Center. Retrieved 2014-03-31.
- ↑ Stu Burro (September 13, 2006). Asteroids. Cleveland, Ohio USA: Case Western Reserve University. Retrieved 2014-03-31.
- ↑ Larry McNish (March 20, 2014). RASC Calgary Centre - Planetary Orbits. Calgary, Alberta, Canada: The Royal Astronomical Society of Canada. Retrieved 2014-03-31.
- 1 2 Takashi Ito; Kiyotaka Tanikawa (October 2002). "Long-term integrations and stability of planetary orbits in our Solar system". Monthly Notice of the Royal Astronomical Society 336 (2): 483-500. doi:10.1046/j.1365-8711.2002.05765.x. http://adsabs.harvard.edu/abs/2002MNRAS.336..483I. Retrieved 2014-03-31.
- ↑ Robert Grant Aitken; Eric Doolittle (1932). New general catalogue of double stars within 120 of the North pole. Publication 417. Washington, D.C.: Carnegie institution of Washington. Bibcode:1932ADS...C......0A. Retrieved 2014-04-02.
- ↑ H. M. Jeffers; W. H. Van Den Bos; F. M. Greeby (1963). "Index catalogue of visual double stars, 1961.0". Publications of the Lick Observatory 21 (1). http://cdsbib.u-strasbg.fr/cgi-bin/cdsbib?1963IDS...C......0J. Retrieved 2014-04-04.
- ↑ J. Dommanget (March 1983). "Un catalogue des composantes d'etoiles doubles et multiples (C.C.D.M.)". Bulletin d'Information du Centre de Donnees Stellaires (24): 83-90. http://cdsbib.u-strasbg.fr/cgi-bin/cdsbib?1983BICDS..24...83D. Retrieved 2014-04-04.
- ↑ J. Dommanget; O. Nys (1994). "Catalogue des composantes d'etoiles doubles et multiples (CCDM) premiere edition - Catalogue of the components of double and multiple stars (CCDM) first edition". Com. de l'Observ. Royal de Belgique (115): 1. http://cdsbib.u-strasbg.fr/cgi-bin/cdsbib?1994CoORB.115....1D. Retrieved 2014-04-04.
- ↑ C. E. Worley; G. G. Douglass (November 1997). "The Washington Double Star Catalog (WDS, 1996.0)". Astronomy & Astrophysics, Supplement Series 125 (1): 523. doi:10.1051/aas:1997239. http://cdsbib.u-strasbg.fr/cgi-bin/cdsbib?1997A%26AS..125..523W. Retrieved 2014-04-04.
- ↑ Brian D. Mason; Gary L. Wycoff. William I. Hartkopf; Geoffrey G. Douglass; Charles E. Worley (December 2001). "The 2001 US Naval Observatory double star CD-ROM. I. The Washington double star catalog". Journal of Astronomy 122 (6): 3466-71. http://cdsbib.u-strasbg.fr/cgi-bin/cdsbib?2001AJ....122.3466M. Retrieved 2014-04-04.
- 1 2 Sue Lavoie (January 10, 2005). PIA07218: Tiny Particles, So Far Away. Pasadena, California USA: California Institute of Technology. Retrieved 2014-04-04.
- ↑ J. H. M. M. Schmitt; L. Golub; F. R. Harnden Jr.; C. W. Maxson; R. Rosner; G. S. Vaiana (March 1, 1985). "An Einstein Observatory X-ray Survey of Main-Sequence Stars with Shallow Convection Zones". The Astrophysical Journal 290 (03): 307-20. doi:10.1086/162986. http://adsabs.harvard.edu/abs/1985ApJ...290..307S. Retrieved 2014-04-04.
- 1 2 3 4 Jon Voisey (March 24, 2011). Companion Stars Could Cause Unexpected X-Rays. Universe Today. Retrieved 2014-04-04.
- 1 2 3 4 5 6 7 8 9 10 11 K. Topka; D. Fabricant; F. R. Harnden Jr.; P. Gorenstein; R. Rosner (April 15, 1979). "Detection of Soft X-rays from α Lyrae and η Bootis with an Imaging X-ray Telescope". The Astrophysical Journal 229 (04): 661-8. doi:10.1086/157000. http://adsabs.harvard.edu/abs/1979ApJ...229..661T. Retrieved 2014-04-04.
- ↑ Charles W. Engelke; Stephan D. Price; Kathleen E. Kraemer (December 2010). "Spectral Irradiance Calibration in the Infrared. XVII. Zero-Magnitude Broadband Flux Reference for Visible-to-Infrared Photometry". The Astronomical Journal 140 (6): 1919-28. doi:10.1088/0004-6256/140/6/1919. http://adsabs.harvard.edu/abs/2010AJ....140.1919E. Retrieved 2014-04-05.
- 1 2 3 Richard H. Munro; Bernard V. Jackson (May 1, 1977). "Physical Properties of a Polar Coronal Hole from 2 to 5 R⊙". The Astrophysical Journal 213 (05): 874-5, 877-86. doi:10.1086/155220. http://adsabs.harvard.edu/abs/1977ApJ...213..874M. Retrieved 2014-04-05.
- 1 2 G. S. Vaiana; J. P. Cassinelli; G. Fabbiano; R. Giacconi; L. Golub; P. Gorenstein; B. M. Haisch; F.R. Harnden Jr. et al. (April 1, 1981). "Results from an extensive Einstein stellar survey". The Astrophysical Journal 244 (04): 163-82. doi:10.1086/158797. http://adsabs.harvard.edu/abs/1981ApJ...245..163V. Retrieved 2014-04-06.
- 1 2 3 F. Lignières; P. Petit; T. Böhm; M. Aurière (June 2009). "First evidence of a magnetic field on Vega. Towards a new class of magnetic A-type stars". Astronomy and Astrophysics 500 (3): L41-4. doi:10.1051/0004-6361/200911996. http://adsabs.harvard.edu/abs/2009A%26A...500L..41L. Retrieved 2014-04-06.
- 1 2 Pascal Petit (July 26, 2009). Magnetic Field On Bright Star Vega. Science Daily. Retrieved 2014-04-06.
- 1 2 Jessica Donaldson (January 20, 2013). Asteroid belt found in the Vega System. Astrobites. Retrieved 2014-04-04.
- ↑ Heasarc (April 18, 2014). Einstein (HEAO-2). Greenbelt, Maryland USA: NASA GSFC. Retrieved 2014-04-18.
- ↑ Robert Petre (May 14, 2004). ROSAT : The Roentgen Satellite. Greenbelt, Maryland USA: NASA GSFC. Retrieved 2014-04-18.
- 1 2 3 4 5 B. Wargelin; J. Drake (December 23, 2009). Proxima Centauri: The Nearest Star to the Sun. Cambridge, Massachusetts, USA: Harvard-Smithsonian Center for Astrophysics. Retrieved 2014-04-18.
External links
- African Journals Online
- Bing Advanced search
- Google Books
- Google scholar Advanced Scholar Search
- International Astronomical Union
- JSTOR
- Lycos search
- NASA/IPAC Extragalactic Database - NED
- NASA's National Space Science Data Center
- NCBI All Databases Search
- Office of Scientific & Technical Information
- PubChem Public Chemical Database
- Questia - The Online Library of Books and Journals
- SAGE journals online
- The SAO/NASA Astrophysics Data System
- Scirus for scientific information only advanced search
- SDSS Quick Look tool: SkyServer
- SIMBAD Astronomical Database
- SIMBAD Web interface, Harvard alternate
- Spacecraft Query at NASA.
- SpringerLink
- Taylor & Francis Online
- Universal coordinate converter
- Wiley Online Library Advanced Search
- Yahoo Advanced Web Search
{{Chemistry resources}}
{{Charge ontology}}
{{Geology resources}}
{{History of science resources}}
{{Principles of radiation astronomy}}
{{Reasoning resources}}