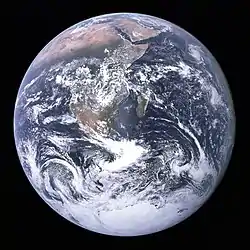
Seven billion humans are now eating, drinking, and living their lives on our magnificent planet. We each require land for our homes, businesses, and recreation. In addition, arable land is used to grow crops to feed us and animals graze on pastures lands where they grow until we eat them. Land is mined to extract a variety of materials including minerals, metals, and the fossil fuels we have used to power our lives for the past 150 years and land is used to store our various waste materials. Forest regions generate oxygen, grow wood and other forest products, sequester carbon, and provide habitats for earth’s remarkable biodiversity made up of millions of unique species, each providing ecosystem services. Ice held in the arctic regions reflects sunlight to cool the planet and sequesters water to maintain the present sea level. Mountain regions grow glaciers, propel rivers and streams, provide awe inspiring vistas, and are unique recreational environments. Clean fresh water provides the essential life substance of humans, animals, and plants—including all that is harvested for our food. Oceans teem with plant and animal life that makes up most levels of the complex food web. Oceans also sequester more than a quarter of the carbon of the planet, keeping it out of the atmosphere and regulating the earth’s climate. Energy on our planet ultimately comes from the sun’s radiation incident on our earth. This energizes photosynthesis in primary producers at the foundation of the food web, as well as the energy accumulated over millions of years as fossil fuels. The sun also directly provides solar power and indirectly provides wind energy.
Every human requires water, consumes food and energy, and produces sewage and other waste—we each have an ecological footprint. The earth’s human population has more than doubled since 1960 requiring twice as much food, more than twice as much energy, and generating at least twice as much waste as only 50 years ago. What are the limits to this growth? When will we reach the carrying capacity of the earth? When will our planet run out of land and fertile soil to grow food, clean fresh water to drink, forests to shelter habitats and sequester carbon, fish in the sea, minerals and fuels to consume, and places to dump our trash?
Although the universe may be infinite, planet earth is definitely finite. This course will help us understand, acknowledge, and plan to live within these limits to increase the well-being of all.
![]() |
Completion status: this resource is considered to be complete. |
![]() |
Attribution: User lbeaumont created this resource and is actively using it. Please coordinate future development with this user if possible. |
The objectives of this course are to:
- Explore the specific limits to growth established by the finite extent of our planet,
- Learn from mistakes made in overlooking these limits and successes from adhering to them,
- Introduce concepts of system analysis, and system thinking,
- Analyze earth as a finite system,
- Understand overshoot, its consequences and mitigation opportunities.
- Study the implications of these limits on planning, system design, and public policy,
- Suggest solutions from a global perspective.
This course is part of the Applied Wisdom Curriculum.
If you wish to contact the instructor, please click here to send me an email.
Text books recommended, but not required for this course are:
- Meadows, Donella H.; Randers, Jorgen; Meadows, Dennis L. (2004). Limits to Growth: The 30-Year Update. Chelsea Green. p. 368. ISBN 978-1931498586.
- A Synopsis Limits to Growth, the 30-year update, by Donella Meadows, Jorgen Randers, Dennis Meadows .
- Brown, Lester R. (2009). Plan B 4.0: Mobilizing to Save Civilization. W. W. Norton & Company. p. 384. ISBN 978-0393337198.
- Available on-line from the Earth Policy Institute.
Sizing Up Planet Earth
We can begin by establishing the quantitative extent and limits of the resources now available on our planet. Because the definitions and extent of each resource is difficult to estimate, a variety of sources are consulted for each. This gives an indication of the range and accuracy of the estimates, and introduces the student to a rich set of data sources.
Calculating limits to growth requires many conversions between equivalent, or near equivalent quantities of land area, energy, emissions, and other quantities. Refer to the course page summarizing Constants, Conversions, and Equivalence Factors for help in these conversions.
- The total Land Area of the earth is 51.51 million square miles, or equivalently 132.5 million square kilometers. [1]
- Arable Land Area
- Annual-crop land area of the earth (all countries, dependencies, and territories) is 5.215 million square miles, or equivalently 13.51 million square kilometers or 1.35 billion hectars. [1]
- Growing crops requires the most productive land of all. The Food and Agriculture Organization (FAO) estimates that today about 1.5 billion hectares of cropland exist worldwide— 1.3 billion hectares of cultivated crops and 0.2 billion hectares of unharvested land that supports temporary pastures and fallow land, failed plantings, and shoulders, shelterbelts, and other uncultivated patches [2] derived from the original FAOSTAT source. [3]
- World cereal (crop) production was 2,285.7 million metric tons in 2008/2009 [4]
- World Grain Production, 1960-2010 has increased steadily since 1960, while the total area harvested has grown less rapidly over the same period of time, indicating a steady increase in world grain yield. Production and consumption have grown at similar rates throughout this period. [5]
- Grazing animals require pasture lands. The FAO defines permanent pasture, which currently amounts to 3.5 billion hectares, as ‘‘land used permanently (five years or more) for herbaceous forage crops, either cultivated or growing wild (wild prairie or grazing land)’’[2] derived from the original FAOSTAT source.[3]
- Forested Land Area area of the earth (all countries, dependencies, and territories) is 14.89 million square miles, or equivalently 38.56 million square kilometers or 3.86 billion hectars. [1]
- According to the FAO’s Forest Resource Assessment (FRA) 2000, there are 3.8 billion hectares of natural forests or plantations worldwide, which experienced an annual deforestation rate of 0.2% between 1990 and 2000. [2]
- Accessing the FAOSTAT 2008 database, world-wide forest area is 4.04 billion hectares.[3] with these attributes, reported in [6]
- Extent of Forest, 2010:
- Total World Forest Area: 4,033,060,000 Hectare
- Percent of Land Area: 31%
- Area per 1000 people: 579 Hectare
- Annual Change Rate:
- 1900-2000: -8,323,000 Hectare, a -0.2% decrease
- 2000-2010: -5,211,000 Hectare, a -0.1% decrease
- Extent of Forest, 2010:
There are approximately 1,386 million cubic kilometers of water on the planet. Almost all of it is salt water. [7]
- The Total Fresh Water Volume on earth is 35 million cubic kilometers. [1]
- Available fresh water from lakes, soil moisture, air humidity, marshes, wetlands, rivers, plant and animals totals 135,000 cubic kilometers
- Available fresh water from groundwater totals 10.6 million cubic kilometers. Much of this is non-renewable
- Unavailable fresh water in glaciers, snow, ice and permafrost totals 24.5 million cubic kilometers.
- Precipitation on salt water is 458,000 cubic kilometers per year
- Fossil Fuel Reserves:
- A credible estimate for the world’s oil resources is 2 trillion barrels [9] of which approximately one half has been consumed.
- Mineral Reserves
- Incident Solar Energy falling on the earth (all countries, dependencies, and territories) is 8.45 billion kilowatt hours per year. [1]
- It is estimated that the total (photoautotrophic) primary production for the Earth was 104.9 Gt C yr−1.[10] Of this, 56.4 Gt C yr−1 (53.8%), was the product of terrestrial organisms, while the remaining 48.5 Gt C yr−1, was accounted for by oceanic production. This establishes the global photosynthetic ceiling.
The Earth's biosphere produces many useful biological products for humans, including (but far from limited to) food, wood, pharmaceuticals, oxygen, and the recycling of many organic wastes. The land-based ecosystem depends upon topsoil and fresh water, and the oceanic ecosystem depends upon dissolved nutrients washed down from the land.[11] Humans also live on the land by using building materials to construct shelters. In 1993, human use of land is approximately:
Land use | Arable land | Permanent crops | Permanent pastures | Forests and woodland | Urban areas | Other |
---|---|---|---|---|---|---|
Percentage | 13.13% [12] | 4.71%[12] | 26% | 32% | 1.5% | 30% |
The estimated amount of irrigated land in 1993 was 2,481,250 km2.[12]
World Population
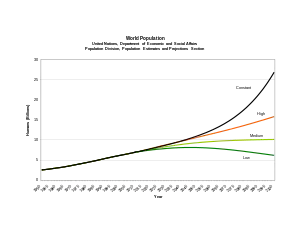
People drive many of the flows that consume stocks and fill sinks toward their limits. More people need more food which requires more land and water to grow. More people buy more goods which require more materials and energy to manufacture, transport and use. People dispose of wastes that require landfills and other sinks to store, dilute, absorb, or transform. Human behaviors such as conservation practices and technology advances can reduce these flows.
The Ehrlich equation (I=P×A×T) estimates the impact of human activity (I) as the product of three factors: the size of the population (P), its level of affluence (A) expressed in income per person, and a technology factor (T), which measures the average impact of each dollar spent.
The history and outlook for world human population is a primary input to any model of earth as a finite dynamic system. What is the likely outlook?
Whereas tens of thousands of years passed before our species reached the one billion mark, around 1800 AD, it took only 130, 33, 15, 13 and 12 years to add each succeeding billion.[13] Unconstrained population growth is an example of exponential growth. Exponential growth starts out slowly and then eventually accelerates at an astonishing rate. US scholar Albert Bartlett pointed out the difficulty people have in grasping the ramifications of exponential growth, stating: "The greatest shortcoming of the human race is our inability to understand the exponential function."[14]
The United Nations Department of Economic and Social affairs estimates and projects world population figures. Their most recent report, World Population Prospects, the 2010 Revision is available on-line. The report considers several scenarios for projecting future world population figures. These are called the medium, low, high, and constant fertility projections The low fertility projection levels off at about 8 billion people in year 2050. The other scenarios continue to grow beyond 2050.
These results are shown in the graph on the right, derived from the report's database.
Assignment
Part 1: Read the World Population Prospects, the 2010 Revision report.
Part 2: Based on the report along with your own experiences and judgments, what factors do you believe are most important for controlling the birth rate, life expectancy, and death rate?
Part 3: Estimate, based on the report, your own experience and judgments when the world population will reach its peak, and the population level at which it will peak.
Ecological footprint
The ecological footprint is a measure of human demand on the Earth's ecosystems. It compares human demand with planet Earth's ecological capacity to regenerate. It represents the amount of biologically productive land and sea area needed to regenerate the resources a human population consumes and to absorb and render harmless the corresponding waste.
In their 2002 paper “Tracking the ecological overshoot of the human economy” a research team reported on their work to account for humanity’s use of the global biosphere. They assessed humanity’s demands and earth’s biological capacity in the areas of: growing crops, grazing animals, harvesting timber, fishing, accommodating infrastructure, and fossil fuel and nuclear energy use. They reported their overall results in terms of the “number of earths” required to sustain the present ecological demands of humanity. Total use exceeded one complete earth in about 1980 and has increased steadily since then. In 1999, the latest year analyzed, the demand was 20% above the earth’s total regenerative ecological capacity.
The WWF Living Planet Report computes the Ecological Footprint and the Water Footprint of Production separately for each country and for the world as a whole. The Ecological Footprint includes these components:
- Carbon Uptake Footprint—Calculated as the amount of forest land required to absorb CO2 emissions from burning fossil fuels, land-use change and chemical processes, other than the portion absorbed by oceans.
- Grazing land Footprint—Calculated from the area used to raise livestock for meat, dairy, hide and wool products.
- Forest Footprint—Calculated from the amount of lumber, pulp, timber products and fuel wood consumed by a country each year.
- Fishing Grounds Footprint—Calculated from the estimated primary production required to support the fish and seafood caught, based on catch data for 1,439 different marine species and more than 268 freshwater species
- Cropland Footprint—Calculated from the area used to produce food and fiber for human consumption, feed for livestock, oil crops and rubber
- Built-up-land Footprint—Calculated from the area of land covered by human infrastructure, including transportation, housing, industrial structures, and reservoirs for hydropower
The global ecological footprint in 2007 was twice the size it was in 1966. The footprint grew to exceed the earth’s annual biocapacity during the 1970s. The world has been in ecological overshoot—what economists call a bubble—since then. People used the equivalent of 1.5 planets in 2007 to support their activities. Consumption flows exceeded creation flows by a factor of 1.5. Stocks are being depleted and sinks are being saturated to make up the difference.
In addition to ecological footprint calculations for individuals, the Global Reporting Initiative (GRI) produces one of the world's most prevalent standards for sustainability reporting for organizations.
Assignment
Part 1: Read The WWF Living Planet Report
Part 2: Based on the information in the report, your own experiences, and your own circumstances and living habits, estimate the size of your own ecological footprint. Use the WWF Footprint calculator, the Global Footprint Network calculator or some other ecological footprint calculator chosen from this gallery of footprint calculators to obtain another estimate of your own ecological footprint
Part 3: Multiply your answer to Part 2 by the today’s world population. How does this compare to the capacity of the earth. Repeat this using a population projection for the year 2050.
Part 4: If your answer in Part 3 above exceeds the capacity of the earth, identify changes you can make in your own lifestyle to shrink your footprint to fit within your share of the earth's capacity. Use the readyourmeter.org website to track your home energy use.
For Further Reading:
Students who would like to learn more calculating ecological footprints may enjoy reading these books:
- Berners-Lee, Mike (2011). How Bad Are Bananas?: The Carbon Footprint of Everything. Greystone Books. p. 256. ISBN 978-1553658313.
- Höhne, Niklas; Phylipsen, Dian; Moltmann, Sara (2007). Factors underpinning future action, 2007 update (PDF). Archived from the original (PDF) on 2007-07-01.
- INVENTORY OF U.S. GREENHOUSE GAS EMISSIONS AND SINKS: 1990 – 2009, EPA 430-R-11-005. U.S. Environmental Protection Agency. 2011.
System Behaviors
Please read the short story “Spending Strategies.” It will be used to illustrate the System Dynamics concepts of stocks, flows, overshoot, and collapse.
A stock is the quantity of some resource present at some particular point in time. In the story, the principal—the amount of accumulated capital—is a stock. Ecological stocks include forest land, fresh water, fertile soils, minerals, and many other natural assets. A stock may represent either a source or a sink. A source is a supply of goods, such as farm land, forest land, or mineral reserves. A sink is a repository for consuming, absorbing, or annihilating some substance. Examples include landfills used for disposing of solid waste, or the atmosphere, used to dilute or transform various air pollutants.
A flow is an on-going change that either increases or decreases the stock. In the story the investment income is a flow that increases the stock (principal) and the annual withdrawals from the account is a flow that decreases the stock.
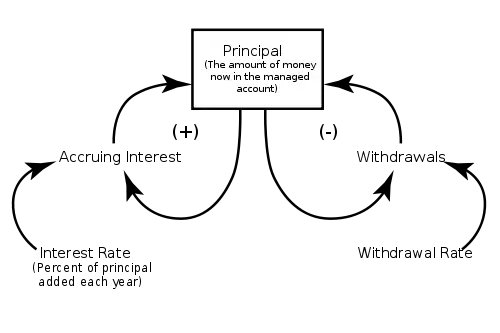
The system structure is illustrated in the diagram shown on the right. Here the stock is shown in an enclosed rectangle. This represents the principal in this example. The feedback loop on the left illustrates the flow into the principal from the accruing interest. This is a positive feedback loop, indicated by the (+) symbol in the loop. The flow depends on the amount of principal available and the interest rate. The feedback loop on the right illustrates the flow out of the principal from annual withdrawals. This is a negative feedback loop, indicated by the (-) symbol. This flow depends on the withdrawal rate and (possibly) the available principal.
The four brothers each illustrate four outcomes that can occur for a stock in a dynamic system (see page 158, figure 4-9 of “Limits to Growth”).
Thrifty Tom ensured the outflow was always less than the income flow and enjoyed continuous growth at a sustainable level. He can continue to withdraw funds at the 4% rate indefinitely and the principal continues to grow. Continuous growth results if the physical limits are essentially infinite or they are themselves growing faster than outflows reduce them. This model is relevant to Sustainable forest management, for example, if: 1) trees are replanted as quickly as they are harvested, 2) the aggregate growth rate of the planted trees (measured for example by board feet) is at least as great as the harvested trees, 3) other consumed stock, such as fertile soil, water, forest habitat, and harvesting resources, are also sustained, 4) no distinction is made between old-growth forest and new growth, and 5) biodiversity is maintained.
Shrewd Sigmund used a more dynamic strategy to approximate a sigmoid growth curve. This can result if information describing the physical limits of the stock and rate of all flows is accurately and instantly available, and this information is accurately, promptly, and correctly responded to. This is rarely achieved within the complexities of ecosystems. Management of fishing stocks attempts to achieve this by adjusting catch limits consistent with on-going monitoring of fish populations. The results are imperfect because the many flows that lead to increasing and decreasing the fish population are complex and impossible to measure accurately and instantly.
Binging Bill spent more than the income flow for an extended period of time. The principal was significantly eroded, however, beginning in year 40 he decreased his spending rate to 4%, and the principal could then begin to recover. Because the principal was nearly exhausted, restoral will take an extremely long time. Eventually, however, the principal can be fully recovered and the cycle could continue. This may lead to an oscillation around the growth limit. This system response is typical when signals or actions are delayed. This model is relevant to recovering an endangered species such as the bald eagle. In the United States the bald eagle population diminished so that by the 1950s there were only 412 nesting pairs in the 48 contiguous US states. Vigorous conservation efforts have allowed the populations to begin to rebound.
Ben’s Bender depleted the principal to the point of collapse. He continued to spend more than the income for 45 years and then finally adjusted the spending rate to equal the income rate. The principal is now so small it is essentially impossible for it to grow back to its original size. The system has collapsed and cannot be recovered in any practical time span. This system response is typical when signals or actions are delayed beyond the point where recovery can ever be achieved. This model is relevant to species extinctions and other ecological events.
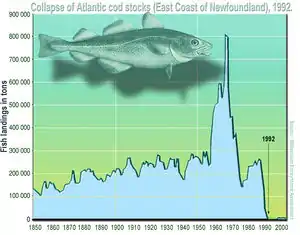
Bill’s oscillation example and Ben’s collapse example illustrate the concept of overshoot. Delays in reacting to a depleting stock reduce that stock to a level where it can not be fully restored in any practical time span. Overshoot comes from delays in feedback. If availability of stock rather than replenishment rates are used to set consumption rates, overshoot will occur. This is probably happening to fertile soils, clean water supplies, forests, many species, and other unique stocks making up our ecology. Sinks—environments for absorbing wastes—can become saturated in the same ways sources can be depleted. We rely on the atmosphere, waterways, and oceans to dilute and absorb pollutants. The capacity of these sinks is finite and may be stressed to overshoot.
Instances of Overfishing provide several real-world ecological examples of overshoot. The collapse of the cod fishery off Newfoundland,[15] and the 1992 decision by Canada to impose an indefinite moratorium on the Grand Banks, is a dramatic example of the consequences of overshoot in the fishing industry. Note, in the diagram to the right, that the collapse occurs soon after the most productive years.
Cod fishing in Newfoundland is also an example of a well-studied system archetype called the tragedy of the commons. Here each fisherman draws down a common stock of fish. Each fisherman earns more money by capturing more fish. However, depleting the common stock of fish harms the overall prospects for fishing next year. The stock will collapse if no mechanism is in place to limit the total number of fish caught. This simple simulation game, is an instructive classroom exercise. The tragedy of the commons is an accurate model of fishing, pollution, water management, forestry and many other ecological systems. It often leads to overshoot before an effective regulation method is put in place. The tragedy of the commons can quickly lead to an unstable equilibrium if evidence or awareness of a future shortage leads to hoarding or other accelerated consumption.
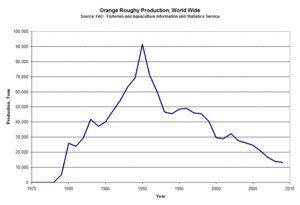
Commercial fishing of Orange Roughy provides another example of environmental overshoot. Referring to the graph at the right, the species was ignored commercially until 1979. Then the world wide catch grew quickly hitting a peak of more than 90,000 tons in 1990 then dropping sharply ever since then. The lifecycle of the Orange Roughy helps explain this trend. The fish have an unusually long life span, typically living more than 100 years. The oldest was verified to be 149 years old. Also, sexual maturity is reached late in life, between the ages of 23 and 40 years. [16] The resilience of the species is very low, minimum population doubling time is more than 14 years. [17] As a result, the production flow—birth of new fish—is quite slow; new fish are born only after their parents live more than 23 years. The catch harvested during the first 15 years of commercial fishing depleted a stock that had been previously undisturbed by fishing and had grown in numbers over a very long period of time. This fish stock was depleted much faster than it could reproduce and even if it was left undisturbed it may take several decades to recover towards its 1975 level. Because of severe overfishing the species has been listed as threatened by the Australian Government in 2006.[18]
The system behaviors described here, including: stocks, flows, feedback, overshoot, Exponential Growth, and the tragedies of the commons, are all elements of the Earth systems engineering and management discipline.
This video dramatizes the possible consequences of growing into overshoot.
Assignments
Part 1: Choose a stock to study that occurs within an ecological system. This may be a source or a sink. Using your own experience and references available to you, list the flows that contribute to the growth of this stock. List the flows that contribute to its depletion.
Part 2: Draw the feedback loop structure diagram for this stock.
Part 3: Estimate the level of this stock over an extended period of time. Chart this as a graph. Extend this graph into the future based on assumptions you make concerning the rate of the flows affecting this stock. Do you predict continuous growth, sigmoid growth, overshoot and oscillation, or overshoot and collapse of this resource?
Part 4: Study the figure charting the collapse of the Atlantic cod stocks on the east coast of Newfoundland, concentrating on the history through 1968. Imagine you were a Newfoundland cod fisherman in 1968. What prediction would you have made at that time, based on the history of catches to date, for the quantity of fish to be caught over the next 20 years? Would you have suggested any change in fish management policies? Explain why or why not. Now read the article "Twilight of the Cod". Based on this information, what prediction would you have made for the quantity of fish to be caught over the next 20 years? Would you have suggested any change in fish management policies? Explain why or why not.
Part 5: Why were the feedback mechanisms in place at the time insufficient to prevent overshoot and collapse? Are the feedback mechanisms now in place sufficient?
Part 6: Analyze the overfishing examples described above as examples of tragedies of the commons. What solution, if any, was in place to protect the commons? Why did it fail? Are the present solutions sufficient to preserve the commons?
Sustainability
Recognizing we have a responsibility to conserve and preserve natural resources for the use and enjoyment by future generations is an enduring idea. This is the concept of sustainability.
Seven generation sustainability is an ecological concept that urges the current generation of humans to live sustainably and work for the benefit of the seventh generation into the future. This requires a planning horizon that looks ahead perhaps 175 years.
This idea emerges from the great binding law of the Iroquois nations, which states:
“ | In all of your deliberations in the Confederate Council, in your efforts at law making, in all your official acts, self interest shall be cast into oblivion. Cast not over your shoulder behind you the warnings of the nephews and nieces should they chide you for any error or wrong you may do, but return to the way of the Great Law which is just and right. Look and listen for the welfare of the whole people and have always in view not only the present but also the coming generations, even those whose faces are yet beneath the surface of the ground – the unborn of the future Nation. | ” |
More recently, in 1987, the World Commission on Environment and Development defined a sustainable society is one that:
“ | meets the needs of the present without compromising the ability of future generations to meet their own needs. | ” |
The precautionary principle (or precautionary approach) is often used as a rational for environmental preservation, including sustainability. It states that if an action or policy has a suspected risk of causing harm to the public or to the environment, in the absence of scientific consensus that the action or policy is harmful, the burden of proof that it is not harmful falls on those taking the action.
Ecologist and Economist Herman Daly established the following conditions [19] for achieving a sustainable system:
- Renewable resources are used no faster than they can be replenished. Examples include fertile soil, clean water, forest, crops, and fish.
- Nonrenewable resources are used no faster than sustainable renewable substitutes are developed. Examples include fossil fuels, minerals, and ancient groundwater, known as fossil water.
- The rates of pollution emission do not exceed the ability of the environment to assimilate the waste. Examples include the biodegradation rate of various wastes such as municipal solid waste.
Because these conditions are rarely met, we are borrowing from the future to live as we do.
Sustainable Agriculture
Food Security—ensuring access to adequate food for all—and Environmental Protection—preserving and sustaining our environment—are two essential and often distinct goals that are best managed with an integrated approach. This section examines the tensions between these two goals as we study soil depletion, water management, and the world food supply.

Soil Depletion
Fertile soil is a mixture of mineral and organic constituents supporting a complex ecosystem. Fertile soil is created slowly as bedrock weathers, organic materials collect, chemical changes take place, and a soil ecosystem forms. Soil degradation can occur quickly through nutrient depletion, contamination, desertification, erosion, or salination.
Soil depletion occurs when the components which contribute to fertility are removed and not replaced, and the conditions which support soil fertility are not maintained. This leads to poor crop yields. In agriculture, depletion can be due to excessively intense cultivation and inadequate soil management.
Plants require light, water, air, and nutrients including the macronutrients (reactive) nitrogen, phosphorus, and potassium to grow. Nutrients are obtained from fertile soil, which also serves to retain moisture.
Prior to cultivation for agriculture, land regions establish equilibrium where water, nutrients, plant and animal life exits in a steady-state balance. In some areas this equilibrium results in the lush vegetation of a forest. In other dryer areas, a more sparse and fragile balance results in arid grassland such as the high plains region of the United States. Here deep rooted and drought-resistant grasses such as blue grama (Bouteloua gracilis) and buffalograss (Bouteloua dactyloides) draw water from deep within the soil as they serve to hold the soil in place. American bison (buffalo) inhabited the area eating the grasses and replenishing the nutrients in the soil with their manure. Prior to European settlements in the mid 1800s the area was an arid buffalo prairie and essentially unsettled and uninhabited by humans. By the early 1900s settlers hunted the bison nearly to extinction, removed the native grasses and worked to raise cattle and grow farm crops such as wheat, corn, sorghum, and cotton. Rainfall in the region is insufficient to sustain the crops, and the crops were insufficient to retain the soil. The Dust Bowl of the 1930 resulted from this disequilibrium, and its history is a fascinating case study of determined people facing natural limits of the ecological system they inhabited.
Agriculture quickly depletes soil nutrients. Continuing agriculture requires replenishing the soil by importing new fertile soil, moving to new fertile land, or adding nutrients to the soil. Traditionally organic nutrients are added by spreading plant material such as compost or animal manure. A particularly dark, fertile anthropogenic soil found in the Amazon Basin, called Terra preta resulted from one civilization's conscientious work to sustain fertile soil. Present-day agriculture usually adds nutrients by applying synthetic fertilizers containing the required nitrogen, phosphorous, and potassium. Also, atmospheric nitrogen can added to the soil in a process called biological nitrogen fixation by growing legumes usually as part of a crop rotation plan which may introduce a variety of green manure to the soil.
Most approaches to agriculture require consuming non-renewable resources and are therefore not sustainable. Importing fertile soil or moving to new fertile land amounts to disposing of the land after the crops have grown. Applying synthetic fertilizers consumes substantial quantities of fossil fuels (such as natural gas used to manufacture ammonia-based fertilizers) and other minerals, such as the phosphate rock mined from the island nation of Nauru. Synthetic fertilizers also add reactive nitrogen and phosphorous to the environment.
Dirt may be nearly worthless but soil is priceless!
Assignment:
Choose a farm to study, perhaps because it is local, they supply your food, or reliable information about the farm is readily available to you. Understand their approach to soil management. Determine how the fertility of the soil is maintained. Identify the sources of nitrogen, phosphorous, and potassium flows into and out of the soil. Determine how rapidly soil erosion takes place and how it is controlled. What aspects of the soil management are sustainable and which consume non-renewable resources?
For Further Reading:
Students who would like to learn more about the importance of soil and the consequences of its depletion may enjoy reading these books:
- Montgomery, David R. (2008). Dirt: The Erosion of Civilizations. University of California Press. p. 296. ISBN 978-0520258068.
- The Worst Hard Time: The Untold Story of Those Who Survived the Great American Dust Bowl.
- Report of the Great Plains Drought Area Committee, August 27, 1936, Morris Cooke, et al.
- The Plow that Broke the Plains, a 1936 short documentary film.
- A direct link to the film.
- Soil Degradation, A Threat to Developing-Country Food Security by 2020?, by Sara J. Scherr, International Food Policy Research Institute, February 1999
- The Revised Universal Soil Loss Equation, Version 2 (RUSLE2)
Water
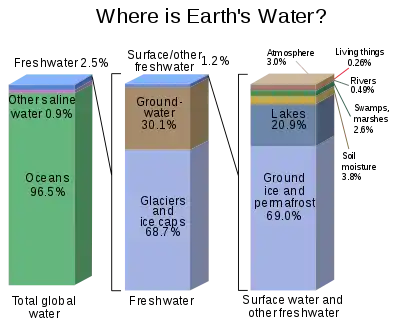
Although Earth appears to have plentiful water supplies, only three per cent of it is fresh rather than salty. Two-thirds of this freshwater is locked up in ice caps and glaciers. Of the remaining one per cent, a fifth is in remote, inaccessible areas and a good deal of the rest arrives when it is least wanted, as monsoonal deluges and floods. The result is that humans are able to make use of only 0.08 per cent of all the world’s water. Water management is the activity of planning, developing, distributing and managing the optimum use of these limited water resources under defined water policies and regulations.
Fresh water is accessible as surface water from streams, rivers, lakes, or reservoirs, or as groundwater from aquifers. While surface water is a renewable resource, recharged from rainfall or snow melt, ground water is often non-renewable. Fossil water is groundwater that has remained sealed in an aquifer for a long period of time. Water can rest underground in fossil aquifers for thousands or even millions of years. When changes in the surrounding geology seal the aquifer off from further replenishing from precipitation, the water becomes trapped within, and is known as fossil water.
Access to fresh water varies by region and by season. While one particular watershed may have a surplus of water, another may be experiencing a drought or other deficit. Because water is difficult to transport in large quantities, water management is best addressed on a regional basis.
Part of the current pressure on water resources comes from increasing demands for animal feed. Meat production requires 8-10 times more water than cereal production.[20]
There is concern that the state of peak water—the point in time when available water will begin a permanent decline—is being approached in many areas around the world. Some areas are suffering from peak renewable water, where entire renewable flows are being consumed for human use, peak non-renewable water, where groundwater aquifers are being overpumped (or contaminated) faster than nature recharges them, and peak ecological water, where ecological and environmental constraints are overwhelming the economic benefits provided by water use.
As one example, the Ogallala Aquifer in the southern high plains of the US is being mined at a rate that far exceeds replenishment—a classic example of peak non-renewable water. Portions of the aquifer will not naturally recharge due to layers of clay between the surface and the water-bearing formation, and because rainfall rates simply do not match rates of extraction for irrigation.[21]
Assignment:
Part 1: Determine the source of the water you use where you live. Is it surface water drawn from a renewable source, replenished from precipitation or is it drawn from an aquifer? If it is drawn from an aquifer does the discharge rate exceed the refresh rates?
Part 2: Choose a farm to study, perhaps because it is local, they supply your food, or reliable information about the farm is readily available to you. Understand their approach to water management. Are the crops rain-fed or irrigated? If the crops are irrigated, what is the source of the irrigation water? Is this renewable or non-renewable?
For Further Reading:
Students who would like to learn more about the importance of fresh water and the consequences of its depletion may enjoy reading these books:
- Black, Maggie; King, Jannet (2009). The Atlas of Water: Mapping the World's Most Critical Resource. University of California Press. p. 128. ISBN 978-0520259348.
- Water for Food, Water for Life: A Comprehensive Assessment of Water Management
- An overview of Aquifer Depletion, January 23, 2010, Encyclopedia of the Earth, by Lester Brown.
- Case study: the history and outlook of the Ogallala Aquifer
- Opie, John (2000). Ogallala: Water for a Dry Land. University of Nebraska Press. p. 477. ISBN 978-0803286146.
- "Since the expansion of irrigated agriculture in the mid-1940s, greater amounts of water have been pumped from the [Ogallala] aquifer than have been recharged. As a result, some areas have experienced water-level declines in excess of 100 feet from predevelopment to 1990." [22]
- "as a whole, the amount of water that has been legally appropriated in the Ogallala is well above the amount of water that is recharged to the aquifer system each year. As such, it can only be expected that the Ogallala Aquifer will continue to be stressed and will continue to decline at varying, albeit, improved levels. With continued declines in the amount of water in storage, it can be only assumed there will be some point in time when the aquifer will not be able to support all water demands placed upon it." [23]
- Case study: the rapid decimation of the Aral Sea
- Snitow, Alan; Kaufman, Deborah (2004). Thirst. DVD
World Food Supply
Is earth’s bounty sufficient to feed the world’s growing population now and into the future? To help answer that question the United Nations Food and Agriculture Organization carefully assembles[24],[25], data, collected from almost every country, on agricultural production. This data is readily available from their FAOSTAT database.
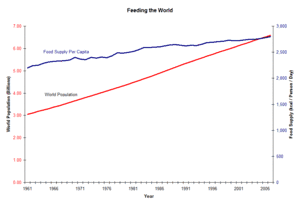
Using data from the FAOSTAT Food Balance Sheets the world food supply, expressed in kilocalories per person per day, is graphed here (in dark blue, use the right-hand scale.) The world population is also graphed, in red, using the left hand scale. Note that as population more than doubled from about 3 billion people in 1961 to more than 6 billion in 2007, the food supply, measured in kilocalories per person per day, actually increased from about 2,200 to nearly 2,800 during that time.
This has been accomplished by steadily increasing the grain yield measured in tons of grain per hectare planted. This is plotted here, based on world grain production data[26]:
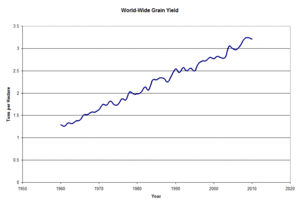
In 1960 world grain production was 824 million tons[27], and it has increased to more than 2,200 million tons in 2010. This is graphed here (in red, use the left-hand scale) along with the number of hectares planed (in blue, use the right-hand scale.)
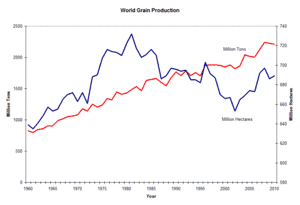
Is everyone well fed? How has this productivity been achieved? How far can it increase? How long can it last? Is it sustainable?
Although sufficient food is available in aggregate, not everyone is getting enough to eat. The World Health Organization cites malnutrition as the gravest single threat to the world's public health. Unfortunately, nearly one billion people are undernourished [28] [29], receiving less than the established minimum dietary energy requirements (MDER). Reasons for this include disasters, underdevelopment, distribution inequities, and regions in continuous crisis.
Increases in food productivity rely, in part, on three unsustainable practices. These are: 1) irrigation, especially using fossil water, 2) soil depletion and 3) land degradation, including mining virgin soil, and use of nitrogen-based fertilizers derived from fossil fuels.
Approximately one fifth of the world’s cropland cannot support agriculture on rainwater alone and must be irrigated using water diverted from rivers, lakes, or aquifers. India alone irrigates 26.5 million hectares using groundwater [30], which is partially non-renewable.
Irrigation impacts the environment by changing the quantity and quality of soil and water and by affecting the natural and social conditions at the tail-end and further downstream of the irrigation scheme.
These impacts result from the changed hydrological conditions caused by the installation and operation of the scheme.
An irrigation scheme often draws water from the river and distributes it over the irrigated area. As a hydrological result it is found that:
- the downstream river discharge is reduced,
- the evaporation in the scheme is increased,
- the groundwater recharge in the scheme is increased,
- the level of the water table rises, and
- the drainage flow is increased.
In addition to these direct effects, effects of irrigation on soil and water quality are also indirect and complex. Water logging and soil salination are part of these, whereas the subsequent impacts on natural, ecological and socio-enonomic conditions is intricate.
Irrigation can also be accomplished by extracting groundwater by wells. This often results in receding water levels. The effects may be water mining, land/soil subsidence, and, along the coast, saltwater intrusion.
Irrigation projects can have large immediate benefits, but the longer term side effects are often overlooked.
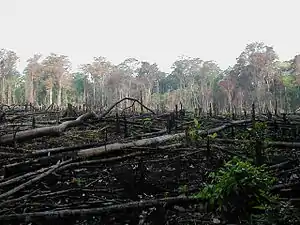
Land degradation is a global problem, largely related to agricultural use. The major causes include:
- Land clearance, such as clearcutting and deforestation,
- Agricultural depletion of soil nutrients through poor farming practices,
- Raising livestock including overgrazing,
- Inappropriate Irrigation and overdrafting,
- Urban sprawl and commercial development,
- Land pollution including industrial waste,
- Vehicle off-roading, and
- Quarrying of stone, sand, ore and minerals.
The main outcome of land degradation is a substantial reduction in the productivity of the land. The major stresses on vulnerable land include:
- Accelerated soil erosion by wind and water,
- Soil acidification and the formation of acid sulfate soil resulting in barren soil,
- Soil alkalinity owing to irrigation with water containing sodium bicarbonate leading to poor soil structure and reduced crop yields,
- Soil salination in irrigated land requiring soil salinity control to reclaim the land,
- Soil waterlogging in irrigated land which calls for some form of subsurface land drainage to remediate the negative effects, and
- Destruction of soil structure including loss of organic matter.
Inorganic fertilizer use has significantly supported global population growth — it has been estimated that almost half the people on the Earth are currently fed as a result of artificial nitrogen fertilizer use.
Unfortunately inorganic fertilizers are now produced in ways which are not sustainable because the resources used in their production are non-renewable. Potassium (K) and phosphorus (P) come from mines (or saline lakes such as the Dead Sea) and such resources are limited. More effective fertilizer use may, however, decrease present usage from mines. Improved knowledge of crop production practices can potentially decrease fertilizer usage of P and K without reducing the critical need to improve and increase crop yields. Atmospheric (unfixed) nitrogen is effectively unlimited (forming over 70% of the atmospheric gases), but this is not in a form useful to plants. To make nitrogen accessible to plants requires nitrogen fixation — conversion of atmospheric nitrogen to a plant-accessible form.
Artificial nitrogen fertilizers are typically synthesized using fossil fuels such as natural gas and coal, which are all limited, non-renewable resources. As an alternative to converting natural gas to syngas for use in the Haber process, it is also possible to convert renewable biomass to syngas (or wood gas) to supply the necessary energy for the process, though the amount of land and resources (ironically often including fertilizer) necessary for such a project may be prohibitive.
Factory farms rely on many unsustainable practices to produce as much meat, eggs, or milk at the lowest possible cost.
Taken together, reliance on these unsustainable resources to boost food production indicates we may already be exceeding and overshooting the sustainable food production capabilities of our planet.
Sea Food
The oceans are so vast they seem limitless. Could we ever deplete the stock of seafood or pollute the oceans? Unfortunately when we look below the surface the answer is yes. We learned about the collapse of the Newfoundland cod industry and the decimation of orange roughy earlier. Ocean acidification is described later in the course. Today at least 75 percent of seafood species are overexploited, fully exploited, or recovering from depletion and need more effective and precautionary management. [31][32] Sustainable seafood guides provide information on which fish species are relatively abundant and which species are overfished.
Seafood supplements land-based agriculture as a source of the world’s food supply. The course page on world fisheries and aquaculture production and utilization provides detailed data on the worldwide seafood catch.
Global total capture and aquiculture production of fish, crustaceans and mollusks continues to increase and reached 142 million tonnes in 2008. While capture has stayed around the 90 million tonnes level since 2001, aquaculture production has continued to show significant growth, increasing at an average annual growth rate of 6.2 percent from 38.9 million tonnes in 2003 to 52.5 million tonnes in 2008. The value of aquaculture production was estimated at USD 98.4 billion in 2008.
China and Peru rank as the top two most productive fishing countries since 1999. In 2007 Indonesia replaced the United States of America in third position.
The most caught species at the global level is by far the anchoveta with about 7.4 million tonnes in 2008. It is followed in ranking by Alaska pollock, Atlantic herring, skipjack tuna and chub mackerel. Growth of tuna fisheries halted in 2008 as catches of this species group decreased by 2.6 percent after the 2007 global record of almost 6.5 million tonnes. The decline of the gadiformes (“Cods, hakes, haddocks”) seems relentless. Shrimp catches have slightly decreased but remained over three million tonnes in 2008. Cephalopod catches set a new record in 2008, although their growth seems to have leveled off.
In 2008, China generated 62 percent of world aquaculture production of fish, crustaceans and molluscs (32.7 million tonnes). Other countries producing over one million tonnes in the same year are India (3.5 million tonnes), Viet Nam (2.5 million tonnes), Indonesia (1.7 million tonnes), Thailand (1.4 million tonnes) and Bangladesh (1 million tonnes).
Carps are the most cultured species in the world with 39 percent of production by volume. Other major groups cultured include shellfishes (oysters, clams, mussels and scallops), other freshwater fish (especially tilapias), shrimps and prawns and salmons.
At single species level, white leg shrimp generated the highest value (USD 9 billion) in 2008, followed by Atlantic salmon (USD 7.2 billion), grass carp (USD 4.8 billion), silver carp (USD 4.8 billion) and other species.
The production of seaweeds and other aquatic plants reached 16.8 million tonnes in 2008, of which aquaculture produced 15.8 million tonnes with a value of USD 7.4 billion. Apart from providing raw materials for industry, aquatic plants are an important food item, especially in Asia.
Total global capture was very steady at about 89.8 million tonnes during the 2006-08 period. Reported inland water catch continued its increasing trend with world capture reaching 10.2 million tonnes in 2008, although the statistics for this sector remain highly approximate for many countries.
Aquaculture production has maintained its steady growth with the average annual growth rate of 6.2 percent for 2003-2008.
About 81 percent of total fishery production (115.1 million tonnes in 2008) was used for direct human consumption. The remaining 19 percent, or 27.2 million tonnes, were destined for non-food products, mainly for the manufacture of fish meal and fish oil. 47.8 percent of the fish destined for human consumption was in live and fresh form. [33]
In 2007, fish accounted for 15.7 percent of the global population’s intake of animal protein and 6.1 percent of all protein consumed. Globally, fish provides more than 1.5 billion people with almost 20 percent of their average per capita intake of animal protein, and 3.0 billion people with 15 percent of such protein. In terms of a world average, the contribution of fish to calories is rather low at 30.5 calories per capita per day (2007 data). However, it can reach 170 calories per capita per day in countries where there is a lack of alternative protein food and where a preference for fish has been developed and maintained (e.g. Iceland, Japan and several small island states).[34]
Assignment:
Part 1: Estimate the number of calories in the food you eat each day. You can use 2,000 calories as a very rough estimate, track the calories you actually eat, or research human nutrition needs to arrive at your estimate.
Part 2: Estimate the fraction of calories you obtain from each of the several high-level food types listed in the food for water section of the conversion summary provided for this course.
Part 3: Based on your estimates above, and the liters / calorie requirements of the foods you eat calculate the liters of fresh water required to grow the food you eat each day.
Part 4: Calculate the hectares of crop land required to grow the food you eat each day. [I need to add data to make this possible.]
Additional Assignment 1:
Part 1: Visit the seafood section of a supermarket or other store in your area.
Part 2: Observe the types of fish they sell. Research these species using the Seafood Watch database, the SeaChoice database, or equivalent advisory lists available for your area. Note that these services provide Smartphone apps that may be convenient for you to use.
Part 3: If any species offered for sale are rated as “avoid” by these advisories, then ask to speak to the seafood manager or store manager. Ask the manager if he or she is aware of the “avoid” rating, and is aware of the issues leading to the “avoid” rating. Ask why they choose to offer these particular fish for sale. Ask if they will consider changing to one of the alternative species listed by the advisory.
Additional Assignment 2:
Part 1: Study aquaculture.
Part 2: Determine the conditions under which aquaculture is a more sustainable approach to providing food than is fish capture from wild fisheries. Under what conditions is it a less sustainable solution? Consider issues of waste handling, side-effects of antibiotics, competition between farmed and wild animals, genetic modification, trophic levels, and using other fish to feed more marketable carnivorous fish.
Additional Assignment 3:
Part 1: Study the International Whaling Commission 1986 moratorium on commercial whaling and their 1994 creation of the Southern Ocean Whale Sanctuary.
Part 2: Investigate the number of whales, dolphins, and porpoises now captured or killed annually. Include the extermination of dolphins at Iki Island, Japan in your investigation.
Part 3: Describe what provisions of the international treaty are working and what is not working. Why?
For Further Reading:
Students who would like to learn more about food production and the consequences of our food and agricultural choices may enjoy reading these books:
- Pollan, Michael (2007). The Omnivore's Dilemma: A Natural History of Four Meals. Penguin. p. 450. ISBN 978-0143038580.
- Danson, Ted; D'Orso, Michael (2011). Oceana: Our Endangered Oceans and What We Can Do to Save Them. Rodale Books. p. 320. ISBN 978-1605292625.
- Greenberg, Paul (2011). Four Fish: The Future of the Last Wild Food. Penguin. p. 304. ISBN 978-0143119463.
- Kurlansky, Mark (1998). Cod: A Biography of the Fish that Changed the World. Penguin. p. 294. ISBN 978-0140275018.
Forests
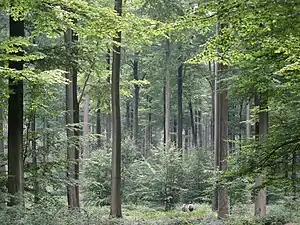
Forests are earth’s greatest living archive. They provide an environment for sustaining and potentially increasing biodiversity. They provide refuge, recreation, food, cultural heritage, and even spiritual inspiration for billions of people.
Forests provide the logs used for lumber and fuel as well as other forest products used as pulp for paper and other products. Forests are also an important source of food, including fruits, camellia (tea), oil seeds, nuts, and bamboo products.
According to the Report of the United Nations Conference on Environment and Development, (Rio de Janeiro, June 3-14, 1992):
“The vital role of all types of forests in maintaining the ecological processes and balance at the local, national, regional and global levels through, inter alia, their role in protecting fragile ecosystems, watersheds and freshwater resources and as rich storehouses of biodiversity and biological resources and sources of genetic material for biotechnology products, as well as photosynthesis, should be recognized.”
Old growth forests represent significantly greater biodiversity than do forest plantations or other younger forests or orchards. In addition, old growth forests sequester significantly more carbon that younger forests.[35]
Less visibly, but perhaps most importantly, forests sequester carbon. The mass of a growing tree accumulates primarily by capturing carbon from the carbon dioxide in the atmosphere. The world’s forests contain more carbon stock than the entire atmosphere. [36]
As part of the carbon cycle, carbon enters into the biomass pool via photosynthesis, and then becomes entrained and cycled through the entire global food chain. Carbon moves out of the biomass pool through decomposition and respiration or through deposition in long-term storage in soil or geologic and fossil deposits. [35] In addition, burning biomass, for example when using wood as a fuel, releases carbon from the biomass, transferring it largely into the atmosphere.
The world's carbon stocks in forests is steadily declining, as this table of total forest carbon stock (in million tonnes) shows:
Year: | 1990 | 2000 | 2005 | 2010 |
---|---|---|---|---|
Carbon in biomass | 299,224 | 293,843 | 291,299 | 288,821 |
Carbon in dead wood | 34,068 | 33,172 | 32,968 | 32,904 |
Carbon in litter | 38,855 | 38,748 | 38,825 | 38,984 |
Carbon in soil | 300,425 | 295,073 | 293,232 | 291,662 |
Total carbon stock | 672,572 | 660,836 | 656,324 | 652,371 |
Source: Global Forest Resource Assessment, 2010, Table 2.25 [36]
The world’s forests store more than 650 billion tonnes of carbon, 44 percent in the biomass, 11 percent in dead wood and litter, and 45 percent in the soil. Globally carbon stocks are decreasing as a result of the loss of forest area; however the carbon stock per hectare has remained almost constant for the period 1990–2010.
In recent years, estimates for deforestation and forest degradation were shown to account for 20-25% of greenhouse gas emissions, higher than the transportation sector. [37] Reducing Emissions from Deforestation and Forest Degradation (REDD) is a set of steps designed to use market and financial incentives to reduce the emissions of greenhouse gases from deforestation and forest degradation.
When wood or paper products are needed, seek products that are certified to come from responsibly managed forests to increase forest sustainability.
Assignment:
Part 1: Visit a local lumber yard or home center. Examine the lumber they have for sale and look for any Forest Certifications for the wood being sold.
Part 2: Learn more about the particular certifications claimed. What organization issued the certification? Is the agency independent or sponsored by a logging company? What are their requirements for awarding the certification? Has any old growth forest been harvested, damaged, or disturbed to obtain this wood? Can you determine the forest where the wood was harvested? Is that forest sustainably managed?
Part 3: Is the certified wood any more expensive or lower quality than the uncertified wood?
For Further Reading:
Students who would like to learn more about forest preservation may enjoy reading these books:
- Myths & Facts… Forests, Carbon, and Global Warming, a PowerPoint presentation by Doug Heiken, Oregon Wild
- Dietrich, William (1993). The Final Forest: The Battle for the Last Great Trees of the Pacific Northwest. Penguin. p. 304. ISBN 978-0140177503.
- Robbins, Jim (2012). The Man Who Planted Trees: Lost Groves, Champion Trees, and an Urgent Plan to Save the Planet. Spiegel & Grau. p. 240. ISBN 978-1400069064.
Ecosystem Services
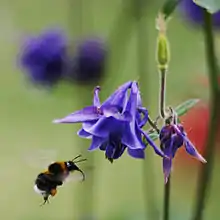
We all benefit from a multitude of resources and processes that are supplied by natural ecosystems. Collectively, these benefits are known as ecosystem services and include essential products like clean drinking water and processes such as the decomposition of wastes.
In 2004 the United Nations formalized definitions of ecosystem services in the Millennium Ecosystem Assessment, a four-year study involving more than 1,300 scientists worldwide.[38] This report grouped ecosystem services into four broad categories:
- Provisioning services, such as the production of food and water including:
- food (including seafood and game), crops, wild foods, and spices
- water
- pharmaceuticals, biochemicals, and industrial products, and
- energy (including hydropower, and biomass fuels)
- Regulating services, such as the control of climate and disease, including:
- carbon sequestration and climate regulation
- waste decomposition and detoxification
- purification of water and air
- pest and disease control
- Supporting services, such as nutrient cycles and seed dispersal including:
- nutrient dispersal and cycling
- crop pollination,
- seed dispersal, and
- primary production
- Cultural opportunities, such as spiritual and recreational benefits, including:
- cultural, intellectual and spiritual inspiration
- recreational experiences (including ecotourism), and
- scientific discovery
Although these services are essential, we rarely account for their value in our economic models. For example, pollination of crops by bees is required for 15-30% of U.S. food production. If sufficient numbers of wild bees are available this can be accomplished “for free” – that is, outside of traditional financial accounting. However if wild bee populations are displaced or destroyed, then these crops will be lost, or some costly substitute to wild bee pollination will have to be found. In either case the economic costs, as traditionally recognized by financial accounting systems, would be substantial. The recently published British National Ecosystem Assessment estimates the value to Britain of pollinating insects at £430m and the worth of all British ecological services in the billions of dollars. Another study estimates the value of ecosystem services world-wide at approximately $33 trillion per year. [39]
Maintaining or increasing biodiversity is a widely accepted strategy for preserving and sustaining the wide variety of these essential ecosystem services. Unfortunately species are being lost at a considerable rate, perhaps more quickly than any previous time in human history.
These concerns lead a group of the world’s scientists to write this warning in 1992[40]:
"The irreversible loss of species, which by 2100 may reach one-third of all species now living, is especially serious. We are losing the potential they hold for providing medicinal and other benefits, and the contribution that genetic diversity of life forms gives to the robustness of the world's biological systems and to the astonishing beauty of the earth itself. Much of this damage is irreversible on a scale of centuries, or permanent. Other processes appear to pose additional threats. Increasing levels of gases in the atmosphere from human activities, including carbon dioxide released from fossil fuel burning and from deforestation, may alter climate on a global scale. Predictions of global warming are still uncertain — with projected effects ranging from tolerable to very severe — but the potential risks are very great."
The World Wide Fund for Nature (WWF) is an international non-governmental organization working on issues regarding the conservation, research and restoration of the environment. They publish the Living Planet Index in a report designed to measure the status and trends of biodiversity, world wide.
Assignment:
Part 1: Observe and identify the ecosystem services you rely on throughout a particular 3-day-long period of time. Write a list of these services.
Part 2: Estimate the economic benefit you derive from these services. If each were not available, what would you substitute for them?
Part 3: Select one ecosystem service from the list created in part 1. Identify and list the various species that contribute to that service.
Additional Assignment:
Part 1: Study the Whitebark Pine, Pinus albicaulis.
Part 2: Identify the ecosystem services provided by the Whitebark pine. Identify the various species that depend on this tree for part of their life cycle.
Part 3: Identify the threats to the Whitebark Pine and steps that could be taken to preserve it.
For Further Reading:
Students who would like to learn more about ecosystem services may enjoy reading these books:
- [find some to suggest]
- The World Wildlife Fund, Living Planet Report — a science-based analysis on the health of our planet and the impact of human activity.
- Put a value on nature!, TED Talk, Pavan Sukhdev, December 2011
Nonrenewable Sources
Non-renewable resources are natural resources which cannot be produced, grown, generated, or used on a scale which can sustain its consumption rate, once used there is no more remaining. These resources often exist in a fixed amount and are consumed much faster than nature can create them.
Fossil Fuels
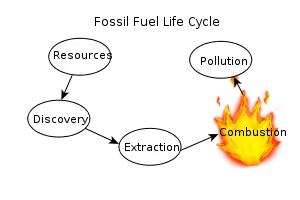
Fossil fuels are fuels formed by natural resources such as anaerobic decomposition of buried dead organisms. The fossil fuels, which contain high percentages of carbon, include coal, petroleum, and natural gas. Fossil fuels store energy collected from sunlight (via photosynthesis in the original organisms when they were living) gathered over millions of years.
Fossil fuels are non-renewable resources because they take millions of years to form, and reserves are being depleted much faster than new ones are being made. The life cycle of fossil fuels is simple, as illustrated here. Earth’s Resources including crude oil, natural gas, or coal are discovered, extracted, and eventually burned. The combustion transforms the fuel into heat and pollutants, including carbon dioxide, nitrogen oxides, sulfur dioxide, smoke, and ash.
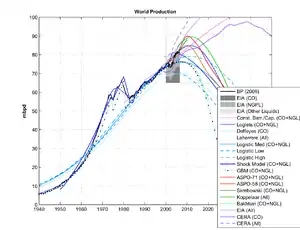
Peak oil is the point in time when the maximum rate of global petroleum extraction is reached, after which the rate of production enters terminal decline. The timing of peak oil is difficult to determine precisely. The graph on the right shows several models, many of which show the peak occurring near the year 2010. According to the International Energy Agency, oil production has already peaked in 2006.
It is likely that we are past the peak of oil production world-wide. Supplies of natural gas and coal are likely to last longer. Although the fossil fuel age will be only a short blip on the timeline of human history, it may leave a long-lasting legacy.
It is difficult to predict if fossil fuel use will be limited by the source—our ability to discover and extract the fuels—or the sink—the earth’s capacity to absorb the pollutants, especially the greenhouse gas emissions. For example, in the United States, more than 90% of greenhouse gas emissions come from the combustion of fossil fuels.[41]
Petrochemicals
Although not fuels, several widely used products derived from petroleum are called petrochemicals. These include ethylene, its many derivatives, inorganic fertilizers, and many plastics.
Assignment:
Part 1: Identify the fossil fuels you use in a typical week. Consider housing (e.g. heating, hot water, laundry, cooking), Transportation (e.g. gasoline, jet fuel, public transportation), and electricity.
Part 2: Identify the petrochemicals you use in a typical week. Consider automotive fluids, plastics, cleansers, and pharmaceuticals. Describe how these are disposed of.
Part 3: Suggest renewable alternatives for each of the uses identified in Parts 1 and 2.
For Further Reading:
Students who would like to learn more about fossil fuel supply may enjoy reading these books:
- Deffeyes, Kenneth S. (2008). Hubbert's Peak: The Impending World Oil Shortage. Princeton University Pres. p. 232. ISBN 978-0691141190.
- Over the Hump, October 2011, blog posting by Leland R. Beaumont
- Gelpke, Basil; McCormack, Ray (2006). A Crude Awakening: The Oil Crash. DVD
- Haney, Bill (2010). The Last Mountain. DVD
Materials
Many materials extracted from the earth are non-renewable resources. These include ores used to extract metals, industrial minerals, masonry materials, and minerals incorporated into a wide variety of products.
The rate at which minerals concentrate into ore bodies or oil pools is slow compared to the pace of human history.[42] Moreover because of the unusual geologic conditions required for their formation or concentration, they are unevenly distributed around the world.
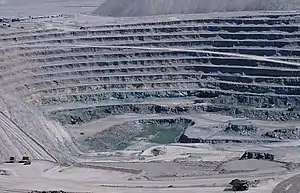
Copper can be studied as one example of mineral extraction and use. Most copper ore is mined or extracted as copper sulfides from large open pit mines in porphyry copper deposits that contain 0.4 to 1.0% copper. This requires huge quantities of earth to be removed to uncover ore sufficient to meet the demands for copper metal.
Research conducted by the USGS quantifies the unwanted by-products associated with copper production. The conversion of one kilogram of copper concentrate from its in-ground condition (ores) into economic service generates an average landscape footprint comprised of 210 kg of mine waste, 113 kg of mill tailings, 2 kg of slag, and 2.3 kg of sulfur-bearing co-product. The corresponding air releases per kg of copper include 0.5 kg of carbon dioxide and 0.2 kg of sulfur dioxide.[43] As the copper content of the ore continues to decline, more earth must be moved to extract each kilogram of copper
The fraction of copper in active use is steadily increasing and the quantity available on Earth may be barely sufficient to allow all countries to reach developed world levels of copper usage. Copper has been in use at least 10,000 years, but more than 95% of all copper ever mined and smelted has been extracted since 1900. As with many natural resources, the total amount of copper on Earth is vast (around 1014 tons just in the top kilometer of Earth's crust, or about 5 million years worth at the current rate of extraction). However, only a tiny fraction of these reserves is economically viable, given present-day prices and technologies. Various estimates of existing copper reserves available for mining vary from 25 years to 60 years, depending on core assumptions such as the growth rate.
Recycling is a major source of copper in the modern world. Because of these and other factors, the future of copper production and supply is the subject of much debate, including the concept of Peak copper, analogue to Peak Oil and other peak minerals.
Many materials extracted for useful purposes are toxic and need to be carefully handled and disposed of. Toxic metals include lead, mercury, cadmium, and others. More than 1,000 metric tons of mercury continues to be extracted from the earth each year, world-wide. More than 500,000 metric tons have been extracted since 1900. [44]
Poor communities traditionally reclaim and reuse materials because of scarce sources. Rich communities are learning to recycle materials because of scarce sinks.
Assignment:
Part 1: Identify the non-renewable materials you use in a typical week.
Part 2: Select one material to study. Research that material’s life cycle from its original extraction and refinement to its final disposition as waste or recycling.
Part 3: Estimate the energy consumption and waste byproducts associated with the life cycle described above.
For Further Reading:
Students who would like to learn more about materials supply may enjoy reading these books:
- [suggest some]
Waste and its Sinks
"When you say you're going to throw something away — where's 'away?' There is no away." ~ Julia Butterfly Hill [45]
She understands that what we call “throwing something away” is simply moving materials to some other repository. A repository used to store or absorb materials is called a sink—it may be a vacant lot, a landfill, cesspool, wetlands, river, lake, ocean, or the atmosphere. The capacity of any sink is finite; they get full. In this section we examine the characteristics of common waste materials and pollutants and the sinks they are typically dumped into.
Perhaps the 16 months the cargo barge Khian Sea spent wandering the seas searching for a place to dump its 14,000 tons of toxic ash provides a metaphor illustrating the difficulties of waste disposal.
One half to three quarters of annual resource inputs to industrial economies are returned to the environment as wastes within a year. Material outputs to the environment from economic activity in the five industrialized countries in one study range from 11 metric tons per person per year in Japan to 25 metric tons per person per year in the United States. [46]
According to a World Resource Institute report, Material Flows in the United States [47], the per capita Domestic Processed Output—materials that are consumed in the domestic economy and subsequently flow to the domestic environment — were 9.8 metric tons per person in the United States in year 2000. The various databases used in the report are publicly available. The mode of first release of these wastes are summarized in this table, derived from the summary database:
Mode of First Release | Percent (by weight) |
---|---|
Controlled on land as solids | 6.45% |
Controlled on land as liquid or partial solids | 11.53% |
Dispersed on land | 3.10% |
Discharged into water | 0.20% |
Discharged to air point sources | 40.63% |
Discharged to air diffuse sources | 32.54% |
Many paths | 5.56% |
Wastewater
[Identify sources, quantify flow rates (globally and per unit), identify sinks and quantify their capacity and assimilation rates.]
Sewage flow is about 60 gallons per capita per day in England and Wales. [48]
More than 80% of sewage in developing countries is discharged untreated, polluting rivers, lakes and coastal areas. [49]
Every day two million tonnes of sewage and other effluents drain into the world’s waters. [50]
Garbage
[Identify sources, quantify flow rates (globally and per unit), identify sinks and quantify their capacity and assimilation rates.]
Municipal Solid Waste
[Identify sources, quantify flow rates (globally and per unit), identify sinks and quantify their capacity and assimilation rates.]
Industrial Pollution
[Identify sources, quantify flow rates (globally and per unit), identify sinks and quantify their capacity and assimilation rates.]
Toxins
[Identify sources, quantify flow rates (globally and per unit), identify sinks and quantify their capacity and assimilation rates.]
The Love Canal was used from 1942 until 1953 as a dump for 21,000 tons of toxic waste. On April 28, 1953 the land was sold to the Niagara Falls City School District for $1. Two schools and a housing project were subsequently built on the site. Many health problems occurred as a result. For example a survey conducted by the Love Canal Homeowners Association found that 56% of the children born from 1974-1978 had at least one birth defect. The National Research Council noted a study which found that exposed children have an "excess of seizures, learning problems, hyperactivity, eye irritation, skin rashes, abdominal pain, and incontinence" and stunted growth.
On August 7, 1978, United States President Jimmy Carter announced a federal health emergency, called for the allocation of federal funds and ordered the Federal Disaster Assistance Agency to assist the City of Niagara Falls to remedy the Love Canal site.
Eventually, the government relocated more than 800 families and reimbursed them for their homes, and the United States Congress passed the Comprehensive Environmental Response, Compensation, and Liability Act (CERCLA), better known as the Superfund Act, that holds polluters accountable for their damages.
It has been calculated that 248 separate chemicals, including 60 kilograms of dioxin, have been unearthed from the canal. This is one example illustrating the latent and persistent dangers created by toxic wastes. As of November 29, 2010, there were 1280 Superfund sites on the National Priorities List in the United States. And of course toxic wastes are generated world-wide. The Blacksmith Institute report on the World's Worst Pollution Problems describes many toxic waste sites in their report of the world’s worst polluted places.
The difficulties and high costs of sustainably disposing of toxic waste invite disposal through deception, fraud, and exploitation. For example, in 1978 31,000 gallons of toxic PCB-contaminated oil were criminally and deliberately spewed in a three-foot swath in 14 counties along some 240 miles of North Carolina highways and at the Fort Bragg Army Base. [51] In other instances waste from a lead works was dumped in the middle of a Moroccan village and 31 children died from lead poisoning, toxic waste in Malaysia was used to reclaim land, and Kenya accepted solid wastes from foreign ships and unclaimed cargo containing toxic chemicals. [52]
And of course, the extent and details of undocumented cases is unknown.
As an aid to track toxic wastes the Toxics Release Inventory (TRI) is a publicly available database containing information on toxic chemical releases and other waste management activities in the United States. Also, TOXMAP is a geographic information system from the United States National Library of Medicine (NLM) that uses maps of the United States to help users visually explore data from the United States Environmental Protection Agency's (EPA) Toxics Release Inventory and Superfund programs.
Radioactive Waste
[Identify sources, quantify flow rates (globally and per unit), identify sinks and quantify their capacity and assimilation rates.]
Radioactive wastes are classified according to their radition levels as: Low Level Waste, Intermediate Level Waste, High Level Waste, and Transuranic Waste.
High level waste (HLW) is produced by nuclear reactors. It contains fission products and transuranic elements generated in the reactor core. It is highly radioactive and often thermally hot. HLW accounts for over 95% of the total radioactivity produced in the process of nuclear electricity generation. The amount of HLW worldwide is currently increasing by about 12,000 metric tons every year. A 1000-MWe nuclear power plant produces about 27 tonnes of spent nuclear fuel (unreprocessed) every year.[53]
Due to historic activities typically related to radium industry, uranium mining, and military programs, there are numerous sites that contain or are contaminated with radioactivity. In the United States alone, the Department of Energy states there are "millions of gallons of radioactive waste" as well as "thousands of tons of spent nuclear fuel and material" and also "huge quantities of contaminated soil and water."[54]
Solutions to the problem of radioactive waste remain elusive. Plans to store radioactive waste in deep geological repositories are encountering significant technical, environmental, and political obstacles. The waste is typically stockpiled while the search for a disposal method goes on.
Air Pollution
[Identify sources, quantify flow rates (globally and per unit), identify sinks and quantify their capacity and assimilation rates.]
Greenhouse Gas
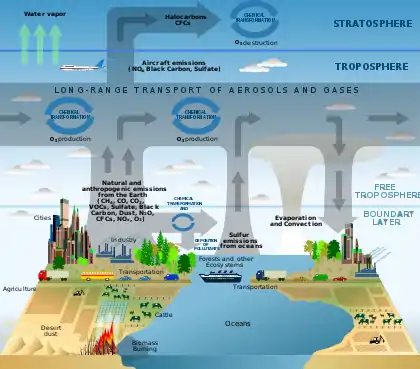
Global greenhouse gas (GHG) emissions have increased steadily since the Industrial Revolution. Since 1990, the reference year used in the Kyoto protocol, emissions have grown at a pace of approximately 1.6 percent a year, from 36 gigatonnes of carbon dioxide equivalents (GtCO2e) in 1990 to 46 GtCO2e in 2005. [55]
The World Resources Institute working paper, World Greenhouse Gas Emissions in 2005, allocates the 2005 world-wide total of 44,153 million metric tons of CO2 equivalents by source sector, end use, and gas in a single information-filled diagram.
The CO2Now organization publishes the current level of atmospheric CO2 levels as measured at the Mauna Loa Observatory. For May 2011, the concentration of CO2 in the atmosphere was 394.39 ppm. In March 1958, when high-precision monitoring began at the Mauna Loa Observatory in Hawaii, atmospheric CO2 was 315.71 ppm. In 1769, the year is which James Watt patented his steam engine, the atmospheric CO2 level was about 280 ppm. To limit the effects of greenhouse gasses on global warming, goals of reducing CO2 to levels 350-500 ppm have been proposed.[56] This increase in atmospheric CO2 concentration contributes directly to global warming.
The carbon capture rates for forest and crop land is given in the conversions page of this course. In addition, Oceans are at present CO2 sinks, and represent the largest active carbon sink on earth, absorbing more than a quarter of the carbon dioxide that humans put into the air.[57] At the present time, approximately one third[58] of human generated emissions are estimated to be entering the ocean. This recent influx of CO2 is causing ocean acidification, and it appears likely that many calcifying species will be adversely affected. These span the food chain from autotrophs to heterotrophs and include organisms such as coccolithophores, corals, foraminifera, echinoderms, crustaceans, and molluscs.
Summary Impact on Sinks
Each cell entry shows the quantity of waste flowing into each sink. If quantitative data is not yet available ● is used to indicate the primary sink and ○ indicates the secondary sink.
Land | Water | Air | |
---|---|---|---|
Wastewater | ○ | ● | |
Garbage | ● | ○ | |
Municipal solid waste | ● | ||
Industrial Pollution | ● | ● | ● |
Toxins | ○ | ● | ○ |
Air Pollution | ● | ||
Greenhouse Gasses | ● | 46 GtCO2e in 2005 |
Assignment:
Part 1: Choose one specific waste material to study in depth.
Part 2: Describe the lifecycle of this material in detail. Identify the sources, flows, stocks, and sinks. Quantify the source and sink flow rates and the rate of assimilation by the various sinks. Also estimate the time interval from extraction or creation of the material until its eventual annihilation or re-use.
Part 3: Describe how this material intersects your life. How do you encounter this material? Do you create it, benefit from it, or use it? Do you discard it or store it? Does it cause any problems or pose any dangers to you?
Further Reading
Students who would like to learn more about waste may enjoy reading these books:
- Gourlay, K.A. (1992). World of Waste: Dilemmas of Industrial Development. Zed Books. p. 246. ISBN 978-0862329884.
- Pollution, an Overview
- Fagin, Dan (2013). Toms River: A Story of Science and Salvation. Bantam. p. 560. ISBN 978-0553806533.
Resource Consumption
Food, water, energy, and materials are all consumed as we live out our lives. Comparing consumption rates to creation flows and available stocks provides an estimate of time to exhaustion of the resource. In addition, waste that results from consumption flows into sinks. In several instances production figures are provided instead of consumption figures. This is because: 1) reliable consumption figures were not available, 2) production anticipates consumption, and 3) production typically consumes the earth's resources.
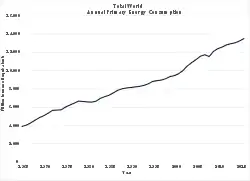
- Detailed world agricultural production figures, reflecting much of the world food supply, are provided on the world agricultural production page of this course.
- Global total capture and aquiculture production of fish, crustaceans and mollusks reached 142 million tonnes in 2008. See the sea food section of this course for more details.
- Fresh Water: As described earlier, fresh water is best studied as a regional resource, rather than on a global basis. Several countries have hit peaks in their water productions and are now facing a fresh water shortfall. Here is a summary of countries that are now at or near freshwater shortfalls. A more complete list is at: [59]
- World energy consumption: In 2008, total worldwide energy consumption was 474 exajoules (474x1018=132,000 TWh). This is equivalent to an average annual power consumption rate of 15 terawatts (1.504x1013)[60] The graph, shown on the right, is drawn based on updated data[61] and shows the continuing trend. (Use the conversion factors to convert from TOE to other units.) World-wide per capita energy consumption was 1,676 TOE in 2008 (4.6 TOE per person per day) and continuing to trend upwards.
- Minerals
- Phosphorus: The average adult requires approximately one gram of phosphorous daily. To maintain a balanced diet, modern agriculture, which uses phosphorus in fertilizers, requires mining 22.5 kg of phosphate rock per year per person. This mining is consuming the mineral faster than geologic cycles can restore it. In addition, much of the phosphorous used in fertilizers is washed into waterways and leads to eutrophication and dead zones in bodies of water. [62] Worldwide production of phosphate rock (assumed to be roughly similar to the consumption) was 176,000 tons in 2010. The world-wide reserves are estimated at 65,000,000 tons. [63]
- The production rates and estimated exhaustion rates are shown in this table for several selected important minerals.
- Rare Earth Elements Critical to High Technology [to be determined]
Success Stories
There are important examples of when decision makers recognized limits to growth and took bold and effective action to reduce pollution flows, limit overfishing, and reduce the environmental impacts.
- People have lived sustainably on the pacific island of Tikopia for many centuries by paying close attention to the ecological limits of their tiny island.[64]
- The US Ban on DDT
- Acid Rain
- Ozone Depletion and the Montreal Protocol
- The International Whaling Commission 1986 moratorium on commercial whaling and their 1994 creation of the Southern Ocean Whale Sanctuary.
- The Sustainable Fisheries Act of 1996
Assignment:
Part 1: Study at least three of the successful outcomes listed above.
Part 2: Identify elements in common that contributed to the successes.
Part 3: Learning from these successes, how can we become better at living within limits to growth?
Quantifying Limits
Here is a tabulation of many limits that have been discussed, a brief rational for choosing the limit, and a reference to one or more expert opinions on choosing the quantitative value of the limits.
Stock | Rationale | Quantitative Limit | Reference |
---|---|---|---|
Food Production | Universal Food security | > 3,000 calories per day per person | # 1 |
atmospheric CO2 concentration | Limit global warming | < 350 ppm CO2 | # 2 |
Limit global warming and mitigation investments. | < between 445 ppm and 535 ppm CO2 | # 3 | |
Anthropogenic radiative forcing; energy imbalance at Earth’s surface, | Limit global warming | < 1 W m-2 above the pre-industrial level | # 2 |
oceanic aragonite saturation | Limit impacts on coral reefs and associated ecosystems | > 80% of the pre-industrial value of the surface ocean aragonite saturation state of 3.44 (Ωarag) | # 2 |
Atmospheric Ozone levels | Filter ultraviolet radiation from the sun. | No more than a <5% reduction from pre-industrial level of 290 Dobson unit | # 2 |
Anthropogenic fixation of N2 from the atmosphere | Limit Eutrophication | < 35 Million tonne N/yr | # 2 |
Anthropogenic flow of Phosphorus | Reduce ocean anoxic events | <10 times the natural background weathering flux of P | # 2 |
Rate of Biodiversity Loss | Maintain ecosystem services | < 10 Extinctions / Million species-years | # 2 |
Global freshwater use | Maintain ecosystems | < 4,000 km3/yr of consumptive blue water use | # 2 |
Fossil Water use | Preserve a non-renewable resource | to last 1,000 years | # 1 |
Fossil Fuel use | Preserve a non-renewable resource | to last 1,000 years | # 1 |
Land-System Change | Maintain ecosystems, and biodiversity and sequester carbon | < 15% of the global ice-free land surface should be converted to cropland | # 2 |
Aerosol Loading | Maintain earth’s radiation balance. | Limit pending further study. | # 2 |
Chemical Pollution | Maintain human and ecosystem health | Limits for some substances have been established, Limits for many other substances is pending further study. | # 2 |
Table References:
The numbers in the last column of the table correspond to these papers:
- This Course
- Rockström, J., W. Steffen, K. Noone, Å. Persson, F. S. Chapin, III, E. Lambin, T. M. Lenton, M. Scheffer, C. Folke, H. Schellnhuber, B. Nykvist, C. A. De Wit, T. Hughes, S. van der Leeuw, H. Rodhe, S. Sörlin, P. K. Snyder, R. Costanza, U. Svedin, M. Falkenmark, L. Karlberg, R. W. Corell, V. J. Fabry, J. Hansen, B. Walker, D. Liverman, K. Richardson, P. Crutzen, and J. Foley. 2009. Planetary boundaries: exploring the safe operating space for humanity. Ecology and Society 14(2): 32. [online] URL: http://www.ecologyandsociety.org/vol14/iss2/art32/
- IPCC Fourth Assessment Report
Creative Solutions
Living within the limits to grow established by our finite planet requires a variety of creative solutions. Here are some to consider:
Applying today's technology:
- Reduce the world’s human population by voluntarily reducing the birth rate.
- Enhance the accuracy of our financial accounting systems by placing a financial value on ecosystem services and an financial cost on pollution, including emission of greenhouse gas. Internalize externalities.
- Supplement traditional financial measures with the Genuine Progress Indicator, the Human Development Index, the Gross National Happiness index, and the Good Country Index.
- Seek to increase well-being for all rather than profits for some.
- Become aware of the externalities of any activity, then take action to reduce impact on others from that externality.
- Prevent overshoot by proactively establishing mechanisms to preserve the commons, including clean air, fresh water, fisheries, forests, fertile soil, ecological services, fossil fuels, materials, and pollution sinks.
- Reduce violence and increase peacefulness to reduce resource consumption worldwide.
- Voluntarily reduce consumption of non-renewable resources. Reuse and recycle those that have been previously extracted.
- Voluntarily reduce consumption of renewable resources to a sustainable level. Reuse and recycle those that have been previously extracted.
- Voluntarily reduce creation of waste materials, especially greenhouse gasses.
- Reduce deforestation and increase reforestation by increasing funding and other means, including Reducing Emissions from Deforestation and Forest Degradation (REDD).
- Use biochar to sequester carbon and improve soils.
- Favor companies and other organizations that meet the SA8000 standard for global accountability.
- Increase the size and quantity of Nature Reserves throughout the world.
- Increase use of ecolabling to help consumers make informed choices.
- Only purchase or consume forest products that are certified by the Forest Stewardship Council.
- Only purchase or consume seafood that is certified by the Marine Stewardship Council.
- Encourage leaders within your own religious community to acknowledge limits to growth and to advocate increased stewardship of nature—for the betterment of His creation.
- Reduce use of transportation that consumes fossil fuels. Walk more and drive less. Use videoconferencing to reduce air travel. Take the train rather than drive.
- Eat foods with a lower trophic level — occurring lower on the food chain. Substitute grains, fruits and vegetables for meat, fish, dairy, and poultry.
- Improvements in our food distribution systems are necessary to get the world’s food supply to each of the world’s people. This TED talk by Josette Sheeran describes approaches that can end hunger now.
- McKinsey and Company publishes greenhouse gas abatement cost curves. These studies estimate the financial benefits and costs for a variety of activities that can reduce greenhouse gasses. Many of these activities have positive financial returns. See the cost curve in the current report for a list of these activities. They are more fully described in their report: Pathways to a Low-Carbon Economy, Version 2 of the Global Greenhouse Gas Abatement Cost Curve. McKinsey & Company, 2009
- The Fourth Assessment Report (AR4) of the United Nations Intergovernmental Panel on Climate Change (IPCC), includes a description of mitigation technologies applicable in the short and medium term.
- Find a solution to the stabilization wedge game that stabilizes CO2 concentrations under 500ppm for the next fifty years, using wedges from a variety of different strategies which fit into the stabilization triangle.
- Reduce use (combustion) of coal by: terminating construction of new coal-fired power plants, retiring existing coal-fired power plants, and improving the efficiency of remaining coal-fired power plants.
- Choose to work fewer hours each week. This will reduce commuting effort and energy, reduce consumption, reduce stress, and increase your leisure time.
- Recognize that once human physiological needs are met, Gross Domestic Product (GDP) becomes a poor surrogate measure of human well being. Adjust activities, policies, and expectations to focus on increasing human well being rather than increasing GDP.
- Increase use and effectiveness of Materials and waste exchanges − markets for buying and selling reusable and recyclable commodities.
- Increase use of Integrated Pest Management techniques to reduce agricultural use of pesticides.
- Use compost instead of synthetic fertilizers to enhance the soil and nourish your lawn.
- In this TED talk, Rob Harmon describes how the market can keep streams flowing using an ingenious market mechanism within the context of prior-appropriation water rights to bring back the water. Farmers and beer companies find common ground and willingly cooperate to rehydrate Montana's long-troubled Prickly Pear Creek.
- The Environmental Institute is using integrated biosystems to recycle wastewater into organic fertilizer, safe agricultural and aquaculture products, biofuel, habitat, and clean water. The process enhances flood control, soil renewal and CO2 reduction.
- Pay as you throw is a usage-pricing model for disposing of municipal solid waste. Users are charged a rate based on how much waste they present for collection to the municipality or local authority. The system is based on two guiding principles of environmental policy: the polluter pays principle and the shared responsibility concept.
- Stop eating fish listed as "avoid" or "Red" in the Seafood Watch database, the SeaChoice database, or equivalent advisory lists available for your area
- In his book, Four Fish, author Paul Greenberg suggests these four priorities for sustaining wild fish:
- Reduce fishing efforts significantly—The world fishing fleet is estimated by the United Nations to be twice as large as oceans can support.
- Convert significant portions, perhaps 10 percent, of the ocean ecosystem to no-catch areas to allow wild fish sanctuaries for breeding and growing,
- Provide global protections for species that migrate through international waters and travel along the coasts of many nations and are therefore not effectively protected by individual national policies.
- Protect the bottom of the food chain to avoid upsetting a complex and poorly understood food web.
- Support the Deep Sea Conservation Coalition in their efforts to reduce the environmental impacts of high seas bottom trawling.
- Take the actions suggested by Oceana to help protect the world’s oceans.
Beyond today's technology:
- Advocate development and use of thorium-fueled nuclear reactors, as described in the book Superfuel by Richard Martin.
- The cheap, clean energy promise of Nuclear Fusion continues to be a few decades off.
- Growing meat directly avoids the financial and environmental costs of raising livestock,
- as described in this June 2011 Scientific American article: Inside the Meat Lab.
- as being developed by Memphis Meats.
Assignment:
Part 1: Choose a solution to study from the list above or from some other source.
Part 2: Estimate the impact this solution can have world-wide on limiting growth to sustainable levels.
Part 3: Describe what you will do to advance that solution.
For Further Reading:
Students who would like to learn more about solutions to living within earth's limits may enjoy reading these books:
- Gore, Al (2009). Our Choice: A Plan to Solve the Climate Crisis. Rodale Books. p. 419. ISBN 978-1594867347.
- Or choose the innovative version implemented as an iPad app, and described in this TED talk.
- Living Beyond Our Means, Natural Assets And Human Well-Being, Statement From The Board, Millennium Ecosystem Assessment, 2005
- Jared, Diamond (2005). Collapse: How Societies Choose to Fail or Succeed. Viking Press. p. 592. ISBN 0143036556.
Toward a stable equilibrium
The limits described here ensure that growth cannot continue indefinitely. Rather than on-going growth, what is needed is a stable dynamic equilibrium where consumption is balanced with generation, and waste flows remain below assimilation rates. What would such a balanced equilibrium be like? How many humans can the earth support at equilibrium? How much food, energy, and materials would be consumed? How much waste would be assimilated? Can we learn to embrace prosperity in terms of flourishing rather than opulence? Would it be a prosperous or an austere life?
The materials in the reading list below describe several actual or possible outcomes. Some are pleasant, others are quite unpleasant.
Assignment
Describe the world as you believe (expect, wish) it will be (can be, must be) approximately 500 years from now. Begin by estimating the world population at that time, and then describe what life will be like in that world.
For Further Reading:
Students who would like to explore models for cultures living at a stable equilibrium with the environment may enjoy reading these books, essays, and reports:
- Jackson, Tim (2011). Prosperity Without Growth: Economics for a Finite Planet. Earthscan Publications Ltd. p. 286. ISBN 978-1849713238.
- Tim Jackson's July 2010 TED talk based on his book.
- The UK Sustainable Development Commission report Prosperity without Growth, that formed the basis for the book.
- Jared, Diamond (2005). Collapse: How Societies Choose to Fail or Succeed. Viking Press. p. 592. ISBN 0143036556.
- Earth at One Billion, an essay by Leland Beaumont describing one possible long-term outcome of the decisions we will be making.
- Envisioning the Good Life, The Good Life in a Steady State Economy, by the Center for the Advancement of the Steady State Economy.
- McDonough, William; Braungart, Michael (2013). The Upcycle: Beyond Sustainability--Designing for Abundance. North Point Press. ISBN 978-0865477483. The upcycle challenges us to learn from nature and design products and systems that recycle materials endlessly without degradation while they derive energy from renewable sources.
- Simply Priceless, an essay by Leland Beaumont about consuming less while enjoying life more.
- A Roadmap for a Secure, Low-Carbon Energy Economy, Sarah Landislaw, Kathryn Zyla, Jonathan Pershing, Frank Verrastro, Jenna Goodward, David Pumphrey, Britt Childs Staley, February, 2009, World Resources Institute.
- Heinberg, Richard (2011). The End of Growth: Adapting to Our New Economic Reality. New Society Publishers. p. 336. ISBN 978-0865716957.
- Macdonald, Copthorne (2004). Matters of Consequence. Big Ideas Press. p. 412. ISBN 978-0968961872.
- Wilkinson, Richard; Pickett, Kate (2011). The Spirit Level: Why Greater Equality Makes Societies Stronger. Bloomsbury Press. p. 400. ISBN 978-1608193417.
- And the companion resource site at www.equalitytrust.org.uk
- Elgin, Duane (2010). Voluntary Simplicity: Toward a Way of Life That Is Outwardly Simple, Inwardly Rich. Harper. p. 240. ISBN 978-0061779268.
- The shareable future of cities, a TED talk by Alex Steffen.
- Stiglitz, Joseph E. (2003). Globalization and Its Discontents. W. W. Norton & Company. p. 304. ISBN 978-0393324396.
- Hawken, Paul; et al. (Amory Lovins, L. Hunter Lovins) (2008). Natural Capitalism: Creating the Next Industrial Revolution. Back Bay Books. p. 416. ISBN 978-0316353007.
- Bahro, Rudolph (1994). Avoiding Social and Ecological Disaster: The Politics of World Transformation. Gateway Books. p. 384. ISBN 978-0946551712.
- Towards a New World System, A Cultural Perspective, published in the Proceedings of the First World Culturelink Conference, Zagreb, June 1995.
- Agenda 21 is an action plan of the United Nations related to Sustainable Development. Implementation is now overseen by the UN Commission on Sustainable Development.
- The UN Department of Economic and Social Affairs, Division for Sustainable Development.
- The text of Agenda 21.
- National Status Information, reported by country
- The International Union for Conservation of Nature and Natural Resources (IUCN) is an international organization dedicated to finding "pragmatic solutions to our most pressing environment and development challenges". They publish many books and articles each year on conservation and sustainability topics.
- Various Factor 10 efforts and the Factor 4 report seek to substantially improve resource efficiency.
- The Natural Step is a non-profit organization setting out the system conditions for the sustainability of human activities on Earth.
- YAN Kun(2011). The tendency equation of the total annual energy consumption and its limit value in the United States (Brief annotation of the connection equation(R)), Xi'an: Xi'an Modern Nonlinear Science Applying Institute.
Resources
- Donella H. Meadows; Jorgen Randers; Dennis L. Meadows (2004). Limits to Growth: The 30-Year Update. Chelsea Green. p. 368. ISBN 978-1931498586.
- A Synopsis Limits to Growth, the 30-year update, by Donella Meadows, Jorgen Randers, Dennis Meadows .
- Brown, Lester R. (2009). Plan B 4.0: Mobilizing to Save Civilization. W. W. Norton & Company. p. 384. ISBN 978-0393337198.
- Available on-line from the Earth Policy Institute.
- Brown, Lester R. (2011). World on the Edge: How to Prevent Environmental and Economic Collapse. W. W. Norton & Company. p. 240. ISBN 978-0393339499.
- Available on-line from the Earth Policy Institute.
- Brand, Stewart (2010). Whole Earth Discipline: Why Dense Cities, Nuclear Power, Transgenic Crops, Restored Wildlands, and Geoengineering Are Necessary. Penguin. p. 352. ISBN 978-0143118282.
- Deffeyes, Kenneth S. (2008). Hubbert's Peak: The Impending World Oil Shortage. Princeton University Press. p. 232. ISBN 978-0691141190.
- World Energy Outlook 2010, published by the International Energy Agency.
- The Food and Agriculture Organization of the United Nations.
- EarthTrends Environmental Information from the World Resources Institute.
- The United States Department of Agriculture, Foreign Agriculture Service
- The Long Now Foundation — working to creatively foster responsibility in the framework of the next 10,000 years
- Let the environment guide our development, 2010 TED talk by Johan Rockstrom
- The Stockholm Resilience Centre
- Planetary Boundaries: Exploring the Safe Operating Space for Humanity 2009, Ecology and Society
- Marie Jahoda; K. L. R. Pavitt; H. S. D. Cole; Christopher Freeman (1973). Models of Doom: A Critique of the Limits to Growth. Universe Publishing. p. 244. ISBN 978-0876639054.
- World3
- The Club of Rome — promoting interdisciplinary analysis, dialogue and action on the fundamental, systemic challenges which are determining the future of humanity.
- the State of the World reports issued by the Worldwatch Institute.
- Jackson, Tim (2011). Prosperity Without Growth: Economics for a Finite Planet. Earthscan Publications Ltd. p. 286. ISBN 978-1849713238.
- The World Wildlife Fund, Living Planet Report — a science-based analysis on the health of our only planet and the impact of human activity.
- The 1992 World Scientists' Warning to Humanity.
- CASSE The Center for the Advancement of the Steady State Economy.
- Daly, Herman. 2005. “Economics in a Full World.” Scientific American, September 2005, pages 100-107
- Population, Environment and Development, The Concise Report, 2001, United Nations,Department of Economic and Social Affairs Population Division
- The Human Development Report 2010, provided by the United Nations Development Program.
- Calculating humanity's Ecological Footprint, by the World Wildlife Fund.
- Höhne, Niklas; et al. (Dian Phylipsen, Sara Moltmann) (2007). Factors underpinning future action, 2007 update (PDF). Archived from the original (PDF) on 2007-07-01.
- INVENTORY OF U.S. GREENHOUSE GAS EMISSIONS AND SINKS: 1990 – 2009, EPA 430-R-11-005. U.S. Environmental Protection Agency. 2011.
- Michael Pollan gives a plant's-eye view, TED Talk, March 2007.
- WattzOn is an online tool to quantify, track, compare, and understand the total amount of energy needed to support all of the facets of your lifestyle.
- The Game Plan, A solution framework for the climate challenge.
- David J.C. MacKay. Sustainable Energy – without the hot air. UIT Cambridge, 2008. ISBN 978-0-9544529-3-3.
- Including this quick reference to energy related constants and conversions.
- The other inconvenient truth, Jonathan Foley, TED Talk, October 2010.
References
- 1 2 3 4 5 Wolfram|Alpha knowledgebase, 2011.
- 1 2 3 Tracking the ecological overshoot of the human economy.
- 1 2 3 Food and Agricultural Organization of the United Nations.
- ↑ FAO Crop Prospects and Food Situation, No. 4, December 2010
- ↑ World on the Edge - Food and Agriculture Data - Crops
- ↑ State of the World's Forests, 2011, Food and Agriculture Organization of the United Nations
- 1 2 Black, Maggie; King, Jannet (2009). The Atlas of Water: Mapping the World's Most Critical Resource. University of California Press. p. 128. ISBN 978-0520259348. Pages 20-21
- ↑ Water for Food, Water for Life: A Comprehensive Assessment of Water Management
- ↑ Deffeyes, Kenneth S. (2008). Hubbert's Peak: The Impending World Oil Shortage. Princeton University Pres. p. 232. ISBN 978-0691141190.
- ↑ Field, C.B.; Behrenfeld, M.J.; Randerson, J.T.; Falkowski, P. year=1998. "Primary production of the Biosphere: Integrating Terrestrial and Oceanic Components". Science 281 (5374): 237–240. doi:10.1126/science.281.5374.237. PMID 9657713.
- ↑ Rona, Peter A. (2003). "Resources of the Sea Floor". Science 299 (5607): 673–674. doi:10.1126/science.1080679. PMID 12560541. http://www.sciencemag.org/cgi/content/full/299/5607/673?ijkey=AHVbRrqUsmdHY&keytype=ref&siteid=sci. Retrieved 2007-02-04.
- 1 2 3 Staff (2008-07-24). "World". The World Factbook. Central Intelligence Agency. Retrieved 2008-08-05.
- ↑ Human population explosion, 2010, The Encyclopedia of earth, Theodore L. Steck
- ↑ Bartlett, Albert (2004). The Essential Exponential! For the Future of Our Planet. Center for Science, Mathematics and Computer Education, University of Nebraska-Lincoln. ISBN 0-9758973-0-6. Retrieved 2011-06-15. See also the author's video lecture: Arithmetic, Population and Energy.
- ↑ Kunzig R (1995) "Twilight of the Cod" Discover, April 1995, p. 52.
- ↑ Danson, Ted; D'Orso, Michael (2011). Oceana: Our Endangered Oceans and What We Can Do to Save Them. Rodale Books. p. 320. ISBN 978-1605292625.
- ↑ http://fishbase.org/summary/SpeciesSummary.php?id=334
- ↑ http://fishbase.org/summary/Hoplostethus-atlanticus.html
- ↑ Toward some operational principles of sustainable development, Herman E. Daly, Ecological Economics, 1990, vol. 2, issue 1, pages 1-6
- ↑ Water in a Changing World, The United Nations World Water Development Report 3, Facts and Figures
- ↑ Nativ, Ronit (1992-01). "Recharge into Southern High Plains aquifer—possible mechanisms, unresolved questions". Environmental Geology (Springer Berlin / Heidelberg) 19 (1): 21–32. doi:10.1007/BF01740574. ISSN (Print) 1432-0495 (Online) 0943-0105 (Print) 1432-0495 (Online). http://www.springerlink.com/content/j12m3754246573t8/. Retrieved 2009-01-30.
- ↑ Aquifers of Texas, Texas Water Development Board, Report 345, November 1995 by John B. Ashworth, Geologist and Janie Hopkins, Geologist
- ↑ Assessment of water level decline rates within the Ogallala aquifer Kansas, Kansas Water Office
- ↑ Food Balance Sheets – A Handbook, Food and Agriculture Organization of the United Nations
- ↑ Technical Conversion Factors for Agricultural Commodities, Food and Agriculture Organization of the United Nations.
- ↑ World on the Edge - Food and Agriculture Data – Crops, Earth Policy Institute.
- ↑ World on the Edge - Food and Agriculture Data – Crops, Earth Policy Institute
- ↑ The State of Food Insecurity in the World, presentation, Food and Agriculture Organization of the United Nations.
- ↑ The State of Food Insecurity in the World, report, Food and Agriculture Organization of the United Nations.
- ↑ Black, Maggie; King, Jannet (2009). The Atlas of Water: Mapping the World's Most Critical Resource. University of California Press. p. 128. ISBN 978-0520259348., page 62.
- ↑ http://na.oceana.org/en/about-us/what-we-do/the-problem
- ↑ Overfishing: a threat to marine biodiversity
- ↑ 2008 FAO yearbook, Fishery and Aquaculture Statistics, Food an Agriculture Organization of the United Nations, Rome 2010, ISSN 2070-6057
- ↑ The State of World Fisheries and Aquaculture, 2010, FAO Fisheries and Aquaculture Department, Food and Agriculture Organization of the United Nations, Rome, 2010, ISSN 1020-5489, ISBN 978-92-5-106675-1
- 1 2 “The Straight Facts on Forests, Carbon, and Global Warming”, Oregon Wild Report
- 1 2 Global Forest Resources Assessment, 2010, Main Report, FAO Forestry Paper 163,
- ↑ Myers,Erin C. (Dec 2007). "Policies to Reduce Emissions from Deforestation and Degradation (REDD) in Tropical Forests" (PDF). Resources Magazine: 7. Retrieved 2009-11-24.
- ↑ Millennium Ecosystem Assessment (MEA). 2005. Ecosystems and Human Well-Being: Synthesis. Island Press, Washington. 155pp.
- ↑ Costanza, Costanza; et al. (Ralph d'Arge, Rudolf de Groot, Stephen Farber, Monica Grasso, Bruce Hannon, Karin Limburg, Shahid Naeem, Robert V. O'Neill, Jose Paruelo, Robert G. Raskin, Paul Sutton, & Marjan van den Belt) (1987). The Value of the World's Ecosystem Services and Natural Capital (PDF). Nature. pp. 253–260. Retrieved June 4, 2011.
- ↑ The 1992 World Scientists' Warning to Humanity
- ↑ US EPA.2000. Inventory of U.S. Greenhouse Gas Emissions and Sinks: 1990-1998, Rep. EPA 236-R-00-01. US EPA, Washington, DC, http://www.epa.gov/globalwarming
- ↑ Limits to Exploitation of Nonrenewable Resources, Earl Cook, 1976, Science, volume 191, pages 676-682
- ↑ Flows of Selected Materials Associated with World Copper Smelting, U.S. Geological Survey Open-File Report 2004-1395 by Thomas G. Goonan
- ↑ U.S. Geological Survey, Mercury statistics
- ↑ Disposability Consciousness, youtube video by Julia Butterfly Hill
- ↑ Matthews, Emily; et al. (Christof Amann, Stefan Bringezu, Marina Fischer-Kowalski, Walter Hüttler, René Kleijn, Yuichi Moriguchi, Christian Ottke, Eric Rodenburg, Don Rogich, Heinz Schandl, Helmut Schütz, Ester Van der Voet, Helga Weisz) (2000). The Weight of Nations, Material Outflows from Industrial Economies (PDF). World Resources Institute. p. 135. ISBN 1-56973-439-9. Retrieved June 9, 2011.
- ↑ Rogich, Donald; et al. (Amy Cassara, Iddo Wernick, Marta Miranda) (2008). Material Flows in the United States, A physical Accounting or the U.S. Industrial Economy (PDF). World Resources Institute. p. 41. ISBN 978-1-56973-682-1. Retrieved June 11, 2011.
- ↑ Harrison, Roy (1990). Pollution: Causes, Effects and Control. Royal Society of Chemistry. p. 408. ISBN 978-0851862835.
- ↑ Water in a Changing World, The United Nations World Water Development Report 3, Facts and Figures
- ↑ Living Planet Report 2010, Biodiversity, biocapacity and development, WWF Report 2010
- ↑ North Carolina PCB Archives
- ↑ Gourlay, K.A. (1992). World of Waste: Dilemmas of Industrial Development. Zed Books. pp. 246, 104. ISBN 978-0862329884.
- ↑ Radioactive Waste management
- ↑ U.S. Department of Energy Environmental Management - "
- ↑ Pathways to a Low-Carbon Economy, Version 2 of the Global Greenhouse Gas Abatement Cost Curve. McKinsey & Company, 2009.
- ↑ http://www.350.org/about/science
- ↑ Earth Institute News, Columbia University, Nov. 18, 2009
- ↑ Takahashik T.; S. C. Sutherland; C. Sweeney; A. Poisson; N. Metzl; B. Tilbrook; N. Bates; R. Wanninkhof et al. (2002). "Global sea-air CO2 flux based on climatological surface ocean pCO2, and seasonal biological and temperature effects". Deep Sea Research II 49: 1601–22. doi:10.1016/S0967-0645(02)00003-6.
- ↑ "The World's Water". Pacific Institute. 2008. pp. tables 1 and 2. Retrieved 2009-01-28.
- ↑ "Consumption by fuel, 1965–2008". Statistical Review of World Energy 2009, BP. July 31, 2006. Archived from the original (XLS) on 2009-07-08. Retrieved 2009-10-24.
- ↑ http://www.bp.com/sectionbodycopy.do?categoryId=7500&contentId=7068481
- ↑ Phosphorus: A Looming Crisis, David A. Vaccari, June 2009, Scientific American
- ↑ Phosphate Rock Statistics and Information, USGS
- ↑ Collapse: How Societies Choose to Fail or Succeed
External Links
- Google Map of The Price of Progress: One state's toxic legacy How an American colony became the top Superfund site with a high autism and cancer rate.
- Google Map of Environmental Disasters