A soda lake or alkaline lake is a lake on the strongly alkaline side of neutrality, typically with a pH value between 9 and 12. They are characterized by high concentrations of carbonate salts, typically sodium carbonate (and related salt complexes), giving rise to their alkalinity. In addition, many soda lakes also contain high concentrations of sodium chloride and other dissolved salts, making them saline or hypersaline lakes as well. High pH and salinity often coincide, because of how soda lakes develop.[lower-alpha 1] The resulting hypersaline and highly alkalic soda lakes are considered some of the most extreme aquatic environments on Earth.[1]
In spite of their apparent inhospitability, soda lakes are often highly productive ecosystems, compared to their (pH-neutral) freshwater counterparts. Gross primary production (photosynthesis) rates above 10 g C m−2 day−1 (grams of carbon per square meter per day), over 16 times the global average for lakes and streams (0.6 g C m−2 day−1), have been measured.[2] This makes them the most productive aquatic environments on Earth. An important reason for the high productivity is the virtually unlimited availability of dissolved carbon dioxide.
Soda lakes occur naturally throughout the world (see Table below), typically in arid and semi-arid areas and in connection to tectonic rifts like the East African Rift Valley. The pH of most freshwater lakes is on the alkaline side of neutrality and many exhibit similar water chemistries to soda lakes, only less extreme.

Geology, geochemistry and genesis
In order for a lake to become alkalic, a special combination of geographic, geological and climatic conditions are required. First of all, a suitable topography is needed, that limits the outflow of water from the lake. When the outflow is completely prevented, this is called an endorheic basin. Craters or depressions formed by tectonic rifting often provide such topological depressions. The high alkalinity and salinity arise through evaporation of the lake water. This requires suitable climatic conditions, in order for the inflow to balance outflow through evaporation. The rate at which carbonate salts are dissolved into the lake water also depends on the surrounding geology and can in some cases lead to relatively high alkalinity even in lakes with significant outflow.

Another critical geological condition for the formation of a soda lake is the relative absence of soluble magnesium or calcium. Otherwise, dissolved magnesium (Mg2+) or calcium (Ca2+) will quickly remove the carbonate ions, through the precipitation of minerals such as calcite, magnesite or dolomite, effectively neutralizing the pH of the lake water. This results in a neutral (or slightly basic) salt lake instead. A good example is the Dead Sea, which is very rich in Mg2+. In some soda lakes, inflow of Ca2+ through subterranean seeps, can lead to localized precipitation. In Mono Lake, California and Lake Van, Turkey, such precipitation has formed columns of tufa rising above the lake surface.
Many soda lakes are strongly stratified, with a well-oxygenated upper layer (epilimnion) and an anoxic lower layer (hypolimnion), without oxygen and often high concentrations of sulfide. Stratification can be permanent, or with seasonal mixing. The depth of the oxic/anoxic interface separating the two layers varies from a few centimeters to near the bottom sediments, depending on local conditions. In either case, it represents an important barrier, both physically and between strongly contrasting biochemical conditions.
Biodiversity
A rich diversity of microbial life inhabit soda lakes, often in dense concentrations. This makes them unusually productive ecosystems and leads to permanent or seasonal "algae blooms" with visible colouration in many lakes. The colour varies between particular lakes, depending on their predominant life forms and can range from green to orange or red.[1]
Compared to freshwater ecosystems, life in soda lakes is often completely dominated by prokaryotes, i.e. bacteria and archaea, particularly in those with more "extreme" conditions (higher alkalinity and salinity, or lower oxygen content). However, a rich diversity of eukaryotic algae, protists and fungi have also been encountered in many soda lakes.[3]
Multicellular animals such as crustaceans (notably the brine shrimp Artemia and the copepod Paradiaptomus africanus) and fish (e.g. Alcolapia), are also found in many of the less extreme soda lakes, adapted to the extreme conditions of these alkalic and often saline environments. Particularly in the East African Rift Valley, microorganisms in soda lakes also provide the main food source for vast flocks of the lesser flamingo (Phoeniconaias minor). The cyanobacteria of the genus Arthrospira (formerly Spirulina) are a particularly preferred food source for these birds, owing to their large cell size and high nutritional value.
Microbial diversity surveys and species richness
.jpeg.webp)
In general, the microbial biodiversity of soda lakes is relatively poorly studied. Many studies have focused on the primary producers, namely the photosynthesizing cyanobacteria or eukaryotic algae (see Carbon cycle). As studies have traditionally relied on microscopy, identification has been hindered by the fact that many soda lakes harbour poorly studied species, unique to these relatively unusual habitats and in many cases thought to be endemic, i.e. existing only in one lake.[4] The morphology (appearance) of algae and other organisms may also vary from lake to lake, depending on local conditions, making their identification more difficult, which has probably led to several instances of taxonomic confusions in the scientific literature.
Recently, a number of studies have used molecular methods such as DNA fingerprinting or sequencing to study the diversity of organisms in soda lakes.[4][5][6][7][8] These methods are based on DNA extracted directly from the environment and thus do not require microorganisms to be cultured. This is a major advantage, as culturing of novel microorganisms is a laborious technique known to seriously bias the outcome of diversity studies, since only about one in a hundred organisms can be cultured using standard techniques.[9] For microorganisms, the phylogenetic marker gene small subunit (SSU) ribosomal RNA is typically targeted, due to its good properties such as existence in all cellular organisms and ability to be used as a "molecular clock" to trace the evolutionary history of an organism.[10] For instance, 16S ribosomal RNA gene clone libraries revealed that the bacterial community of the lake with the highest salinity was characterized by a higher recent accelerated diversification than the community of a freshwater lake, whereas the phylogenetic diversity in the hypersaline lake was lower than that in a freshwater lake.[11] Culture-independent surveys have revealed that the diversity of microorganisms in soda lakes is very high, with species richness (number of species present) of individual lakes often rivaling that of freshwater ecosystems.[11]
Biogeography and uniqueness
In addition to their rich biodiversity, soda lakes often harbour many unique species, adapted to alkalic conditions and unable to live in environments with neutral pH. These are called alkaliphiles. Organisms also adapted to high salinity are called haloalkaliphiles. Culture-independent genetic surveys have shown that soda lakes contain an unusually high amount of alkaliphilic microorganisms with low genetic similarity to known species.[5][6][7][8] This indicates a long evolutionary history of adaptation to these habitats with few new species from other environments becoming adapted over time.
In-depth genetic surveys also show an unusually low overlap in the microbial community present, between soda lakes with slightly different conditions such as pH and salinity.[3][7] This trend is especially strong in the bottom layer (hypolimnion) of stratified lakes,[4] probably because of the isolated character of such environments. Diversity data from soda lakes suggest the existence of many endemic microbial species, unique to individual lakes.[3][7] This is a controversial finding, since conventional wisdom in microbial ecology dictates that most microbial species are cosmopolitan and dispersed globally, thanks to their enormous population sizes, a famous hypothesis first formulated by Lourens Baas Becking in 1934 ("Everything is everywhere, but the environment selects").[12]
Ecology
Carbon cycle

Photosynthesis provides the primary energy source for life in soda lakes and this process dominates the activity at the surface. The most important photosynthesizers are typically cyanobacteria, but in many less "extreme" soda lakes, eukaryotes such as green algae (Chlorophyta) can also dominate. Major genera of cyanobacteria typically found in soda lakes include Arhtrospira (formerly Spirulina) (notably A. platensis), Anabaenopsis,[13] Cyanospira, Synechococcus or Chroococcus.[14] In more saline soda lakes, haloalkaliphilic archaea such as Halobacteria and bacteria such as Halorhodospira dominate photosynthesis. However, it is not clear whether this is an autotrophic process or if these require organic carbon from cyanobacterial blooms, occurring during periods of heavy rainfall that dilute the surface waters.[1]
Below the surface, anoxygenic photosynthesizers using other substances than carbon dioxide for photosynthesis also contribute to primary production in many soda lakes. These include purple sulfur bacteria such as Ectothiorhodospiraceae and purple non-sulfur bacteria such as Rhodobacteraceae (for example the species Rhodobaca bogoriensis isolated from Lake Bogoria[15]).
The photosynthesizing bacteria provide a food source for a vast diversity of aerobic and anaerobic organotrophic microorganisms from phyla including Pseudomonadota, Bacteroidota, Spirochaetota, Bacillota, Thermotogota, Deinococcota, Planctomycetota, Actinomycetota, Gemmatimonadota, and more.[1][3] The stepwise anaerobic fermentation of organic compounds originating from the primary producers, results in one-carbon (C1) compounds such as methanol and methylamine.
At the bottom of lakes (in the sediment or hypolimnion, methanogens use these compounds to derive energy, by producing methane, a procedure known as methanogenesis. A diversity of methanogens including the archaeal genera Methanocalculus, Methanolobus, Methanosaeta, Methanosalsus and Methanoculleus have been found in soda lake sediments.[1][16] When the resulting methane reaches the aerobic water of a soda lake, it can be consumed by methane-oxidizing bacteria such as Methylobacter or Methylomicrobium.[1]
Sulfur cycle
Sulfur-reducing bacteria are common in anoxic layers of soda lakes. These reduce sulfate and organic sulfur from dead cells into sulfide (S2−). Anoxic layers of soda lakes are therefore often rich in sulfide. As opposed to neutral lakes, the high pH prohibits the release of hydrogen sulfide (H2S) in gas form. Genera of alkaliphilic sulfur-reducers found in soda lakes include Desulfonatronovibrio and Desulfonatronum.[1] These also play important an ecological role besides in the cycling of sulfur, as they also consume hydrogen, resulting from the fermentation of organic matter.
Sulfur-oxidating bacteria instead derive their energy from oxidation of the sulfide reaching the oxygenated layers of soda lakes. Some of these are photosynthetic sulfur phototrophs, which means that they also require light to derive energy. Examples of alkaliphilic sulfur-oxidizing bacteria are the genera Thioalkalivibrio, Thiorhodospira, Thioalkalimicrobium and Natronhydrogenobacter.[1]
Nitrogen and other nutrients
Nitrogen is a limiting nutrient for growth in many soda lakes, making the internal nitrogen cycle very important for their ecological functioning.[17] One possible source of bio-available nitrogen is diazotrophic cyanobacteria, which can fix nitrogen from the atmosphere during photosynthesis. However, many of the dominant cyanobacteria found in soda lakes such as Arthrospira are probably not able to fix nitrogen.[1] Ammonia, a nitrogen-containing waste product from degradation of dead cells, can be lost from soda lakes through volatilization because of the high pH. This can hinder nitrification, in which ammonia is "recycled" to the bio-available form nitrate. However, ammonia oxidation seems to be efficiently carried out in soda lakes in either case, probably by ammonia-oxidizing bacteria as well as Thaumarchaea.[17]
List of soda lakes
The following table lists some examples of soda lakes by region, listing country, pH and salinity. NA indicates 'data not available':




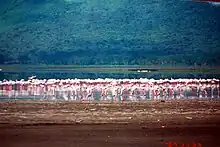
Continent | Name | Country | pH | Salinity |
---|---|---|---|---|
Africa | Wadi El Natrun lakes | Egypt | 9.5 | 5% |
Malha Crater Lake | Sudan | 9.5–10.3 | NA | |
Lake Arenguadi (Green Lake) | Ethiopia | 9.5–9.9[3] | 0.25% | |
Lake Basaka | Ethiopia | 9.6[3] | 0.3% | |
Lake Shala | Ethiopia | 9.8[3] | 1.8% | |
Lake Chitu | Ethiopia | 10.3[3] | 5.8% | |
Lake Abijatta | Ethiopia | 9.9[3] | 3.4% | |
Lake Magadi | Kenya | 10 | >10% | |
Lake Bogoria | Kenya | 10.5 | 35% | |
Lake Turkana | Kenya | 8.5–9.2[18] | 0.25% | |
Lake Nakuru | Kenya | 10.5 | NA | |
Lake Logipi | Kenya | 9.5–10.5 | 2–5% | |
Lake Sonachi (Crater Lake) | Kenya | NA | NA | |
Lake Balangida | Tanzania | NA | NA | |
Lake Manyara | Tanzania | 9.5–10[19] | NA | |
Lake Natron | Tanzania | 9–10.5 | >10% | |
Lake Rukwa | Tanzania | 8–9[19] | NA | |
Lake Eyasi | Tanzania | 9.3[19] | 0.5% | |
Momela Lakes | Tanzania | 9.7 | 22% | |
Lake Ngami | Botswana | |||
Sua Pan | Botswana | 19% | ||
Rombou Lake | Chad | 10.2[20] | 2% | |
Asia | Kartsakhi Lake | Georgia/Turkey | NA | 0.09% |
Kulunda Steppe Lakes | Russia | NA | NA | |
Lake Khatyn | Russia | 10 | NA | |
Lake Van | Turkey | 9.7–9.8 | 2.3% | |
Lake Salda | Turkey | NA | NA | |
Lonar Lake (Crater Lake) | India | 9.5–10.5[5] | 1% | |
Sambhar Salt Lake | India | 9.5 | 7% | |
Khyagar Lake[20] | India | 9.5 | 0.6% | |
Tso Moriri Salt Lake | India | 9.0 | NA | |
Tso Kar Salt Lake | India | 8.8 | NA | |
Surigh Yilganing Kol | Aksai Chin, India/China | NA | NA | |
Tso Tang Lake | Aksai Chin, India/China | NA | NA | |
Aksayqin Hu Lake | Aksai Chin, India/China | NA | NA[21] | |
Lake Hongshan Hu | Aksai Chin, India/China | NA | NA | |
Tianshuihai lake | Aksai Chin, India/China | NA | NA | |
North Tianshuihai lake | Aksai Chin, India/China | NA | NA | |
Kushul lake | India | NA | NA | |
Pangong Salt Lake | India & China | 9.4 | 0.9%[22] | |
Spanggur Tso (Pongur Tso) | India & China | NA | NA | |
Guozha lake | China | NA | NA | |
Qinghai Lake | China | 9.3[23] | 2.2% | |
Namucuo Lake | India | 9.4[23] | 0.2% | |
Lake Zabuye (Drangyer) | China | 10 | NA | |
Torey Lakes | Russia, Mongolia | NA | NA | |
Taboos-nor | Mongolia | NA | NA | |
Europe | Lake Fehér (Szeged) | Hungary | NA | NA |
Böddi-szék | Hungary | 8.8–9.8[24] | NA | |
Lake Neusiedl (Fertő) | Austria, Hungary | 9–9.3[24] | NA | |
Rusanda | Serbia | 9.3[24] | NA | |
Kelemen-szék | Hungary | 9–9.7[24] | NA | |
Malham Tarn | UK | 8.0–8.6[25][26] | NA | |
North America | Mono Lake | US | 9.8[17] | 8% |
Soda Lakes (Nevada) | US | 9.7 | NA | |
Soap Lake | US | 9.7 | 0.7% | |
Baldwin Lake | US | NA | NA | |
Alkali Lake (OR) | US | 11 | NA | |
Summer Lake | US | NA | NA | |
Owens Lake | US | NA | NA | |
Borax Lake | US | NA | NA | |
Manitou Lake | Canada | NA | NA | |
Goodenough Lake | Canada | 10.2 | NA | |
Lake Texcoco | Mexico | 8.8–11.5 | 8% | |
Lake Alchichica | Mexico | 8.9 | NA | |
South America | Antofagasta Lake | Chile | NA | NA |
Australia | Lake Werowrap[20] | Australia | 9.8 | 4% |
Industrial use
Many water-soluble chemicals are extracted from the soda lake waters worldwide. Lithium carbonate (see Lake Zabuye), potash (see lake Lop Nur and Qinghai Salt Lake Potash), soda ash (see Lake Abijatta and Lake Natron), etc. are extracted in large quantities. Lithium carbonate is a raw material in production of lithium which has applications in lithium storage batteries widely used in modern electronic gadgets and electrically powered automobiles. Water of some soda lakes are rich in dissolved uranium carbonate.[27] Algaculture is carried out on a commercial scale with soda lake water.
See also
Notes
References
- 1 2 3 4 5 6 7 8 9 Grant, W. D. (2006). Alkaline environments and biodiversity. in Extremophiles, 2006, UNESCO / Eolss Publishers, Oxford, UK
- ↑ Melack JM, Kilham P (1974). "Photosynthetic rates of phytoplankton in East African alkaline, saline lakes" (PDF). Limnol. Oceanogr. 19 (5): 743–755. Bibcode:1974LimOc..19..743M. doi:10.4319/lo.1974.19.5.0743. Retrieved 27 December 2012.
- 1 2 3 4 5 6 7 8 9 Lanzén A, Simachew A, Gessesse A, Chmolowska D, Øvreås L (2013). "Surprising Prokaryotic and Eukaryotic Diversity, Community Structure and Biogeography of Ethiopian Soda Lakes". PLOS ONE. 8 (8): e72577. Bibcode:2013PLoSO...872577L. doi:10.1371/journal.pone.0072577. PMC 3758324. PMID 24023625.
- 1 2 3 Barberán, A.; Casamayor, E. O. (2010). "Euxinic Freshwater Hypolimnia Promote Bacterial Endemicity in Continental Areas". Microbial Ecology. 61 (2): 465–472. doi:10.1007/s00248-010-9775-6. PMID 21107832. S2CID 6985343.
- 1 2 3 Surakasi, V. P.; Antony, C. P.; Sharma, S.; Patole, M. S.; Shouche, Y. S. (2010). "Temporal bacterial diversity and detection of putative methanotrophs in surface mats of Lonar crater lake". Journal of Basic Microbiology. 50 (5): 465–474. doi:10.1002/jobm.201000001. PMID 20586073.
- 1 2 Dong, H.; Zhang, G.; Jiang, H.; Yu, B.; Chapman, L. R.; Lucas, C. R.; Fields, M. W. (2006). "Microbial Diversity in Sediments of Saline Qinghai Lake, China: Linking Geochemical Controls to Microbial Ecology". Microbial Ecology. 51 (1): 65–82. doi:10.1007/s00248-005-0228-6. PMID 16400537. S2CID 34103123.
- 1 2 3 4 Xiong, J.; Liu, Y.; Lin, X.; Zhang, H.; Zeng, J.; Hou, J.; Yang, Y.; Yao, T.; Knight, R.; Chu, H. (2012). "Geographic distance and pH drive bacterial distribution in alkaline lake sediments across Tibetan Plateau". Environmental Microbiology. 14 (9): 2457–2466. doi:10.1111/j.1462-2920.2012.02799.x. PMC 3477592. PMID 22676420.
- 1 2 Wani, A. A.; Surakasi, V. P.; Siddharth, J.; Raghavan, R. G.; Patole, M. S.; Ranade, D.; Shouche, Y. S. (2006). "Molecular analyses of microbial diversity associated with the Lonar soda lake in India: An impact crater in a basalt area". Research in Microbiology. 157 (10): 928–937. doi:10.1016/j.resmic.2006.08.005. PMID 17070674.
- ↑ Handelsman J (2004). "Metagenomics: application of genomics to uncultured microorganisms". Microbiol Mol Biol Rev. 68 (4): 669–685. doi:10.1128/mmbr.68.4.669-685.2004. PMC 539003. PMID 15590779.
- ↑ Tringe SG, Hugenholtz P (2008). "A renaissance for the pioneering 16S rRNA gene" (PDF). Curr Opin Microbiol. 11 (5): 442–446. doi:10.1016/j.mib.2008.09.011. PMID 18817891. S2CID 10552013.
- 1 2 Wang J (2011). "Do Patterns of Bacterial Diversity along Salinity Gradients Differ from Those Observed for Macroorganisms?". PLOS ONE. 6 (11): e27597. Bibcode:2011PLoSO...627597W. doi:10.1371/journal.pone.0027597. PMC 3220692. PMID 22125616.
- ↑ Baas-Becking, Lourens G.M. (1934), Geobiologie of inleiding tot de milieukunde, The Hague, the Netherlands: W.P. Van Stockum & Zoon
- ↑ Girma, M. B.; Kifle, D.; Jebessa, H. (2012). "Deep underwater seismic explosion experiments and their possible ecological impact – the case of Lake Arenguade – Central Ethiopian highlands". Limnologica - Ecology and Management of Inland Waters. 42 (3): 212–219. doi:10.1016/j.limno.2011.12.002.
- ↑ Zavarzin GA, Zhilina TN, Kevbrin VV (1999). "Alkaliphilic microbial community and its functional diversity". Mikrobiologiya. 86: 579–599.
- ↑ Milford, A. D.; Achenbach, L. A.; Jung, D. O.; Madigan, M. T. (2000). "Rhodobaca bogoriensis gen. nov. And sp. Nov., an alkaliphilic purple nonsulfur bacterium from African Rift Valley soda lakes". Archives of Microbiology. 174 (1–2): 18–27. doi:10.1007/s002030000166. PMID 10985738. S2CID 12430130.
- ↑ Antony CP, Colin Murrell J, Shouche YS (July 2012). "Molecular diversity of methanogens and identification of Methanolobus sp. as active methylotrophic Archaea in Lonar crater lake sediments". FEMS Microbiol. Ecol. 81 (1): 43–51. doi:10.1111/j.1574-6941.2011.01274.x. PMID 22150151.
- 1 2 3 Carini, Stephen A.; Joye, Samantha B. (2008). "Nitrification in Mono Lake, California: Activity and community composition during contrasting hydrological regimes". Limnology and Oceanography. 53 (6): 2546–2557. Bibcode:2008LimOc..53.2546C. CiteSeerX 10.1.1.307.8534. doi:10.4319/lo.2008.53.6.2546. S2CID 1869692.
- ↑ Yuretich, R. F.; Cerling, T. E. (1983). "Hydrogeochemistry of Lake Turkana, Kenya: Mass balance and mineral reactions in an alkaline lake". Geochimica et Cosmochimica Acta. 47 (6): 1099–1109. Bibcode:1983GeCoA..47.1099Y. doi:10.1016/0016-7037(83)90240-5.
- 1 2 3 Hsieh, T. H.; Chen, J. J. J.; Chen, L. H.; Chiang, P. T.; Lee, H. Y. (2011). "Time-course gait analysis of hemiparkinsonian rats following 6-hydroxydopamine lesion". Behavioural Brain Research. 222 (1): 1–9. arXiv:1302.5809. doi:10.1016/j.bbr.2011.03.031. PMID 21435355. S2CID 119601350.
- 1 2 3 Hammer U.T. (1986). Saline lake ecosystems of the world, Kluwer Academic Publishers, Hingham MA
- ↑ "Glacial and lake fluctuations in the area of the west Kunlun mountains during the last 45000 years" (PDF). Retrieved 23 July 2013.
- ↑ T.V. Ramachandra & others. "EIA study near Pangong lake, India". Retrieved 27 December 2012.
- 1 2 Xing, P.; Hahn, M. W.; Wu, Q. L. (2009). "Low Taxon Richness of Bacterioplankton in High-Altitude Lakes of the Eastern Tibetan Plateau, with a Predominance of Bacteroidetes and Synechococcus spp". Applied and Environmental Microbiology. 75 (22): 7017–7025. Bibcode:2009ApEnM..75.7017X. doi:10.1128/AEM.01544-09. PMC 2786500. PMID 19767472.
- 1 2 3 4 Felföldi, T. S.; Somogyi, B. R.; Márialigeti, K. R.; Vörös, L. (2009). "Characterization of photoautotrophic picoplankton assemblages in turbid, alkaline lakes of the Carpathian Basin (Central Europe)". Journal of Limnology. 68 (2): 385. doi:10.4081/jlimnol.2009.385.
- ↑ Bradley, P. (March 2002). White-clawed Crayfish (Austropotamobius pallipes) At Craven Limestone Complex SAC, North Yorkshire (Report). Field Studies Council. Archived from the original on 18 October 2008. Retrieved 19 August 2008.
- ↑ Allan Pentecost (2009). "The Marl Lakes of the British Isles". Freshwater Reviews. 2 (1): 167–197. doi:10.1608/FRJ-2.2.4. S2CID 86157620.
- ↑ "Uranium in Sambhar lake waters in India" (PDF). Retrieved 5 December 2013.